Insulin resistance and high blood pressure -
Reaven, MD, GRECC B , VA Medical Center, Miranda Avenue, Palo Alto, CA Get Permissions. toolbar search Search Dropdown Menu. toolbar search search input Search input auto suggest.
This content is only available via PDF. Copyright © by the American Diabetes Association. View Metrics. Email alerts Article Activity Alert.
Online Ahead of Print Alert. Latest Issue Alert. Online ISSN Print ISSN Books ShopDiabetes. org ADA Professional Books Clinical Compendia Clinical Compendia Home News Latest News DiabetesPro SmartBrief. Resources ADA Professional Membership ADA Member Directory Diabetes.
X Twitter Facebook LinkedIn. In the same setting, tempol normalized insulin resistance in Ang II-infused rats, and enhanced insulin-induced PI3K activation, suggesting that Ang II-induced insulin resistance can be restored by removing the oxidative stress [ 42 ]. A further mechanism that account for role of insulin resistance in the aetiology of hypertension is the upregulation of AT1 receptors induced by hyperinsulinemia.
This potentiates the detrimental effects of Ang II on cardiovascular CV system. Altogether, these observations provide clear insights of the pathogenic mechanisms of Ang II—induced insulin resistance in the development of hypertension.
Molecular mechanisms that account for angiotensin II-induced insulin resistance. The binding of insulin to its own receptor evokes the tyrosine autophosphorylation of the β subunit, which, in turn activates, in sequence, the insulin receptor substrate-1 and phosphatidylinositol 3-kinase green arrows.
This cascade, accounts for the biological effects of insulin. Angiotensin II stimulation, through the activation of NADPH oxidase, stimulates the generation of reactive oxygen species, which, in turn promote the threonine phosphorylation of insulin receptor substrate-1 red arrows.
This induces the proteasome-dependent degradation of insulin receptor substrate-1, interrupting the insulin signalling cascade. Ser, serine; P, phospho; Tyr, tyrosine; ROS, reactive oxygen species; PI3K, phosphatidylinositol 3-kinase; IRS-1, insulin receptor substrate Dysregulation of sympathetic nervous system is a feature of hypertension [ 44 ].
For instance, adipocytes and cardiac myocytes are both target organs of insulin, and mainly express β3 and β1-ARs, respectively. β-ARs stimulation results in an impairment of insulin signalling and insulin-induced glucose uptake, which profoundly differs between the two cell types regarding the kinetic and molecular mechanisms involved.
In particular, β-ARs stimulation in adipocytes impairs insulin signalling within few minutes, whereas, in cardiac myocytes stimulation of β-ARs has a biphasic effect on insulin-stimulated glucose uptake, with an initial additive followed by an inhibitory action.
On the contrary, in cardiac myocytes Akt plays a key role in the cross-talk between β1-ARs and IR. Actually, upon the phosphorylation site, Akt can have a favourable or detrimental effect on insulin signalling and insulin-induced glucose uptake.
In particular, short-term β-ARs stimulation induces Akt phosphorylation in threonine that results in an increase in glucose uptake, whereas long-term stimulation induces Akt phosphorylation in serine and impairs insulin evoked-glucose uptake and insulin-induced tyrosine autophosporylation of IR [ 46 ].
In addition, it has been demonstrated in cardiac myocytes, that chronic Akt activation is sufficient to impair insulin signal by inducing IRS-1 phosphorylation and proteasome-dependent degradation [ 47 ]. LVH, CA, and renal dysfunction are expression of hypertension-evoked TOD, and are independent risk factors for both fatal and nonfatal cardio- and cerebro-vascular events [ 48 , 49 , 50 , 51 , 52 ].
Several studies have shown that insulin resistance promotes the development of left ventricular hypertrophy, carotid atherosclerosis and chronic kidney disease CKD. Development of LVH is a complex and multifactorial process which involves genetic, hemodynamic and anthropometric components, neuro-hormonal stimulation, growth factors and inflammatory mediators [ 53 , 54 , 55 ].
The hemodynamic and metabolic disorders associated with insulin resistance increase the risk LVH. Insulin and insulin-like growth factor IGF -1 are powerful independent determinants of LV mass and geometry in untreated subjects with essential hypertension and normal glucose tolerance [ 56 ].
The presence of both insulin resistance and hypertension in the same patient results in a mixed pattern of cardiac hypertrophy, caused by an elevation in both cardiac preload and afterload [ 57 ].
Experimental data suggest that insulin might be involved in the pathogenesis of both the concentric and eccentric patterns of LVH. Stimulation of myocardial cell growth and activation of the sympathetic nervous system might preferentially lead to concentric LV hypertrophy through a direct trophic effect and pressure overload, whereas sodium and water retention could lead to eccentric LV hypertrophy through volume overload [ 58 ].
The direct effect of insulin on myocardial cell growth could be mediated, at least in part, by the IGF-1 receptors [ 59 ]. In addition, the myocardium in the insulin-resistant individual shows the presence of mononuclear cell infiltration all along the conduction system making the myocardium in the insulin-resistant hypertensive patient an ideal substrate for cardiac arrhythmia and sudden death.
Several epidemiological studies have underlined the role of insulin resistance, hyperinsulinemia in the development of atherosclerosis, and in the pathogenesis of its clinical consequences.
Several mechanisms have been proposed as responsible for these phenomena. These include both direct effects on the arterial wall and indirect actions on lipid and glucose metabolism.
Considerable evidence exists that demonstrate that endothelium as a physiological target of insulin and, consequently, a potential link between insulin resistance and atherosclerosis.
In the endothelium, the stimulation of IRs activates insulin signaling via the PI3-K pathway, leading to NO production. The production of NO by endothelial cells, stimulated by insulin or insulin-like growth factor, leads to a host of anti-inflammatory and antithrombotic effects, which are antiatherogenic.
The anti-inflammatory effects of NO include the decreases of expression of vascular cell adhesion molecule-1, intracellular adhesion molecule-1, and E-selectin and reduced secretion of the proinflammatory cytokines monocyte chemoattractant protein—1 and tumor necrosis factor-α TNF-α.
Interestingly, the insulin-induced antithrombotic effects depends on gene expression regulation, which decreases the platelet adhesion by increasing prostacyclin production [ 60 ]. Of note, the gene encoding for coagulation factor II thrombin receptor, which is involved in the enhanced risk of coronary artery diseases in patients with hypertension [ 61 ], and it has been recently proposed as a marker of clinical conditions characterized by insulin resistance like metabolic syndromes, obesity, T2D, hepatic steatosis, and atherosclerosis [ 62 ].
Atherosclerotic process is regulated by inflammatory mechanisms [ 63 , 64 , 65 ]. Notably, insulin resistance has been recognized as a chronic, low-level, inflammatory state [ 66 ].
Although several mechanisms may contribute to the pathogenesis of hypertension, the NO system appears to play a major role.
NO is an important messenger molecule that plays a critical role in a wide variety of physiological functions, including immune modulation, vascular relaxation, neuronal transmission, and cytotoxicity.
Interestingly, the polymorphism of the promoter of the inducible nitric oxide synthase iNOS gene, NOS2A, revealed a positive association with essential hypertension [ 67 ].
This aspect is linked to insulin resistance because insulin stimulation of glucose uptake in skeletal muscle and adipose tissue is a NO-dependent phenomenon. Moreover, iNOS has been shown to be crucial for the development of insulin resistance [ 68 ]. The role that inflammation plays in atherosclerosis is amplified by the renin-angiotensin system via its effects on adhesion molecules, growth factors, and chemoattractant molecules, which modulate the migration of inflammatory cells into the subendothelial space.
Clinical and experimental data have increased our knowledge on the effects of the Ang II. In particular, it has been documented that Ang II stimulates the production of ROS through its AT1 receptor [ 69 ], resulting in a proinflammatory modulator. The low grade of inflammation is a common feature of atherosclerosis and insulin resistance.
The two major determinants of this state are the pro-inflammatory cytokines, TNF-α and interleukin-6 IL The available experimental data indicate that TNF-α is involved in the pathophysiology of hypertension.
In particular, in vitro, TNF-α stimulates the production of endothelin-1 and angiotensinogen. Moreover, in SHR, TNF-α synthesis and secretion and fat angiotensinogen mRNA are increased in response to lipopolysaccharide LPS stimulation in comparison with non-hypertensive control rats [ 70 ].
At molecular level, TNF-α blocks the action of insulin in cultured cells [ 71 ], though the serine phosphorylation of IRS-1, decreasing the tyrosine kinase activity of the insulin receptor [ 72 ].
The key role of TNF-α in the pathogenesis of hypertension has been also documented in humans. In particular, the TNF-α gene locus seems to be involved in insulin resistance-associated hypertension [ 73 ]. A positive correlation has been found between serum TNF-α concentration and both SBP and insulin resistance [ 74 ].
Up-regulation of TNF-α secretion has also been observed in peripheral blood monocytes from hypertensive patients [ 75 ]. TNF-α also determines endothelial dysfunction linked to insulin resistance [ 76 ].
In contrast, treatment of T2D or obese human subjects with an antibody specific for TNF had no effect on insulin sensitivity [ 78 ]. IL-6 is a multifunctional cytokine produced by many different cell types, including immune cells, endothelial cells, fibroblasts, myocytes, and adipose tissue, which mediates inflammatory as well as stress-induced responses.
It has been documented that BP was a significant and independent predictor of circulating IL-6 concentrations in women but not in men [ 79 ]. A polymorphism in the promoter of the IL-6 gene has also shown divergent associations with BP [ 80 ].
IL-6 stimulates the central nervous system and the sympathetic nervous system, which may result in hypertension [ 81 ].
However, other mechanisms cannot be excluded. IL-6 might increase in parallel to the modification of the redox state of the vascular wall in chronic hypertension, as occurs in some animal models of hypertension [ 82 ].
IL-6 is a well-characterized acute inducer of fibrinogen, which is a determinant of blood viscosity. Finally, IL-6 might increase BP enhancing the expression of angiotensinogen, leading to higher concentration of Ang II. Interestingly, leptin, a cytokine-like molecule increasingly recognized to regulate several inflammatory pathways acting on a receptor of the IL-6 family seems also to be associated with hypertension.
The leptin signal, via central leptin receptors, is believed to interact with the central sympathetic nervous system [ 83 ]. Infusion of leptin leads to increases in BP [ 84 ]. Transgenic mice overexpressing leptin had elevated BP, which is normalized by adrenergic and ganglionic blockade [ 85 ].
Hypertension is believed to contribute to CKD by increasing glomerular capillary pressure, proteinuria, endothelial dysfunction, and sclerosis, leading to nephron damage [ 86 ]. Insulin resistance is associated with activation of both renin-angiotensin system and sympathetic nervous system activities, contributing to increased renal sodium reabsorption, associated fluid retention and hypertension [ 87 ].
Moreover, this state is accompanied by the increased endothelial cells proliferation and intrarenal lipid and hyaluronate deposition in the matrix and inner medulla [ 88 ].
These depositions increase intrarenal pressure and volume in the tightly encapsulated kidney, leading to parenchymal prolapse and urine outflow obstruction, which result in slow tubular flow and subsequently increased sodium reabsorption, especially in the loop of Henle.
These functional and structural changes provoke compensatory lowered renal vascular resistance, elevated kidney plasma flow, glomerular hyperfiltration, and stimulation of renin angiotensin system, despite volume expansion.
In addition, these changes in combination with the raise in BP levels associated with the insulin-resistant state increase the tubular reabsorption and maintain sodium balance. The persistence of these compensatory responses, increasing glomerular wall stress, precipitate gradual nephron loss, glomerulosclerosis and eventually end-stage renal disease.
Glomerulosclerosis in the insulin-resistant kidney is peculiar and characterized by lower rate of nephrotic syndrome, fewer lesions of segmental sclerosis and a greater glomerular size compared with the idiopathic variety. In summary, persistence of insulin resistance and suboptimal control of associated hemodynamic and metabolic abnormalities cause renal injury with functional as well as structural nephron loss contributing to elevated BP, which in turn leads to further renal injury, thereby setting off a vicious circle of events leading to further elevated BP and renal injury.
Microalbuminuria is now established as a modifiable predictor of CV morbidity and mortality [ 52 , 89 ] and is considered a manifestation hypertension-induced TOD. Despite its strong association with CV risk, the exact pathogenic mechanisms that link microalbuminuria to CV diseases remains unknown.
Evidence has been garnered that microalbuminuria is a marker of generalized endothelial dysfunction and consequently a risk factor for CV diseases. In particular, this abnormality has been characterized by the presence of transmembrane leakiness [ 90 ].
In addition, microalbuminuria has also been associated with alterations in hemodynamic and vascular responses. In general, microalbuminuria can be considered as an early marker of generalized hypertension-evoked TOD Fig. Relationship between microalbuminuria and insulin resistance in the continuum of cardiovascular disease.
Microalbuminuria, is clinical marker of activation of the insulin resistance-dependent pathogenic mechanisms involved in the genesis and progression of hypertension and hypertension-induced target organ damage.
Epidemiological data show that hypertension is a risk factor for the development of T2D. The combination of hypertension and T2D increases CV risk and requires a strict control of both BP and glycaemia [ 91 ]. Insulin resistanceis involved in the pathogenesis and progression of hypertension as well as T2D.
The low-grade of inflammation induced by insulin resistance is the principal mechanism that accounts for development of endothelial dysfunction, hypertension-induced TOD, and metabolic abnormalities [ 92 ] Fig.
Altogether these data encourage the adoption of a holistic strategy to prevent the development of insulin resistance. In particular, it is well known that hypocaloric diet, and regular exercise training improve insulin sensitivity [ 93 ]. Both are recommended for primary and secondary CV prevention.
It is noteworthy that consistent body of evidence supports the notion that exercise training is able to modify the epigenetics changes that are involved in the development of insulin resistance [ 24 ]. Insulin resistance is a time-dependent organ and tissue specific phenomenon.
Insulin resistance contributes to the dysregulation of peripheral vascular resistance, resulting in an increase of blood pressure.
In arterial hypertension, insulin resistance participates to the development of target organ damage. The persistence of insulin resistance induces the metabolic abnormalities that account for development of type 2 diabetes.
The increased caloric intake and the sedentary habit are the principal environmental factors that account for the development and progression of insulin resistance.
The dysregulation of renin-angiotensin system plays a pivotal role in the development of insulin resistance; thus, the prevention of incident T2D in patients with hypertension requires the inhibition of RAS with AT1 antagonist or ACE inhibitors [ 94 ]. Finally, in the last decades the beneficial effects on the CV system of different classes of glucose-lowering agents have been reported.
In particular, Glucagon-like peptide-1 receptor agonists GLP-1RAs stimulate insulin release and supress glucagon synthesis. There is growing body of evidence that the GLP-1RAs improve CV outcomes in type 2 diabetes.
In fact, they are recommended as first- and second-choice agents in diabetic patients at high CV risk or with overt atherosclerosis, independently from achievement of glycemic control [ 95 , 96 ].
In conclusion, the prevention of the insulin resistance-induced continuum of CV disease requires an integrated approach which involves non pharmacologic and pharmacologic therapies. Conen D, Ridker PM, Mora S, Buring JE, Glynn RJ. Eur Heart J. PubMed Google Scholar.
Meisinger C, Döring A, Heier M. J Hypertens. CAS PubMed Google Scholar. Kramer CK, von Mühlen D, Barrett-Connor E. Mid-life blood pressure levels and the 8-year incidence of type 2 diabetes mellitus: the Rancho Bernardo study.
J Hum Hypertens. Stahl CH, Novak M, Lappas G, Wilhelmsen L, Björck L, Hansson P-O, Rosengren A. High-normal blood pressure and long-term risk of type 2 diabetes: year prospective population based cohort study of men. BMC Cardiovasc Disord.
Google Scholar. Derakhshan A, Bagherzadeh-Khiabani F, Arshi B, Ramezankhani A, Azizi F, Hadaegh F. Different combinations of glucose tolerance and blood pressure status and incident diabetes, hypertension, and chronic kidney disease. J Am Heart Assoc. PubMed PubMed Central Google Scholar.
Izzo R, de Simone G, Chinali M, Iaccarino G, Trimarco V, Rozza F, et al. Insufficient control of blood pressure and incident diabetes. Diabetes Care. Izzo R, de Simone G, Trimarco V, Gerdts E, Giudice R, Vaccaro O, De Luca N, Trimarco B.
Hypertensive target organdamagepredictsincidentdiabetesmellitus. de Simone G, Wang W, Best LG, Yeh F, Izzo R, Mancusi C, Roman MJ, Lee ET, Howard BV, Devereux RB. Target organ damage and incident type 2 diabetes mellitus: the Strong Heart Study.
Cardiovasc Diabetol. Olefsky J, Farquhar JW, Reaven G. Relationship between fasting plasma insulin level and resistance to insulin-mediated glucose uptake in normal and diabetic subjects.
Scherrer U, Randin D, Vollenweider P, Vollenweider L, Nicod P. J Clin Invest. CAS PubMed PubMed Central Google Scholar. Steinberg HO, Chaker H, Leaming R, Johnson A, Brechtel G, Baron AD. Implications for the syndrome of insulin resistance. Fukuda N, Satoh C, Hu WY, Nakayama M, Kishioka H, Kanmatsuse K.
Endogenous angiotensin ii suppresses insulin signaling in vascular smooth muscle cells from spontaneously hypertensive rats. Lembo G, Napoli R, Capaldo B, Rendina V, Iaccarino G, Volpe M, Trimarco B, Sacca L.
Abnormal sympathetic overactivity evoked by insulin in the skeletal muscle of patients with essential hypertension. Sowers JR. Hypertension, angiotensin ii, and oxidative stress. N Engl J Med. Tabit CE, Chung WB, Hamburg NM, Vita JA. Endothelial dysfunction in diabetes mellitus: molecular mechanisms and clinical implications.
Rev Endocr Metab Disord. Tabit CE, Shenouda SM, Holbrook M, Fetterman JL, Kiani S, Frame AA, Kluge MA, Held A, Dohadwala MM, Gokce N, Farb MG, Rosenzweig J, Ruderman N, Vita JA, Hamburg NM.
Protein kinase C-β contributes to impaired endothelial insulin signaling in humans with diabetes mellitus. Mancusi C, Losi MA, Izzo R, Canciello G, Manzi MV, Sforza A, De Luca N, Trimarco B, de Simone G. Effect of diabetes and metabolic syndrome on myocardial mechano-energetic efficiency in hypertensive patients.
The Campania Salute Network. Mancusi C, de Simone G, Best LG, Wang W, Zhang Y, Roman MJ, Lee ET, Howard BV, Devereux RB. Myocardial mechano-energetic efficiency and insulin resistance in non-diabetic members of the Strong Heart Study cohort.
Ferrannini E, Buzzigoli G, Bonadonna R, Giorico MA, Oleggini M, Graziadei L, Pedrinelli R, Brandi L, Bevilacqua S. Insulinresistance in essentialhypertension. Reaven GM, Lithell H, Landsberg L. Hypertension and associated metabolic abnormalities—the role of insulin resistance and the sympathoadrenal system.
Grunfeld B, Balzareti M, Romo M, Gimenez M, Gutman R. Hyperinsulinemia in normotensive offspring of hypertensive parents. Cheng LS, Davis RC, Raffel LJ, Xiang AH, Wang N, Quinones M, Wen PZ, Toscano E, Diaz J, Pressman S, Henderson PC, Azen SP, Hsueh WA, Buchanan TA, Rotter JI.
Coincident linkage of fasting plasma insulin and blood pressure to chromosome 7q in hypertensive hispanic families. Miyaoka K, Kuwasako T, Hirano K, Nozaki S, Yamashita S, Matsuzawa Y.
Cd36 deficiency associated with insulin resistance. Dos Santos JM, Moreli ML, Tewari S, Benite-Ribeiro SA. The effect of exercise on skeletal muscle glucose uptake in type 2 diabetes: an epigenetic perspective. Lembo G, Iaccarino G, Vecchione C, Rendina V, Trimarco B.
Insulin modulation of vascular reactivity is already impaired in prehypertensive spontaneously hypertensive rats. Dietary Supplementation of Vitamin D Prevents the Development of Western Diet-Induced Metabolic, Hepatic and Cardiovascular Abnormalities in Rats. United European Gastroenterol J.
Lee J, Pilch PF. The insulin receptor: structure, function, and signaling. Am J Physiol. Cong LN, Chen H, Li Y, Zhou L, McGibbon MA, Taylor SI, Quon MJ.
Physiological role of akt in insulin-stimulated translocation of glut4 in transfected rat adipose cells. Mol Endocrinol. Hansen L, Ikeda Y, Olsen GS, Busch AK, Mosthaf L.
Insulin signaling is inhibited by micromolar concentrations of H 2 O 2. Evidence for a role of H 2 O 2 in tumor necrosis factor alpha-mediated insulin resistance.
J Biol Chem. Egawa K, Nakashima N, Sharma PM, Maegawa H, Nagai Y, Kashiwagi A, Kikkawa R, Olefsky JM. Persistent activation of phosphatidylinositol 3-kinase causes insulin resistance due to accelerated insulin-induced insulin receptor substrate-1 degradation in 3t3-l1 adipocytes.
Goldstein BJ, Ahmad F, Ding W, Li PM, Zhang WR. Regulation of the insulin signalling pathway by cellular protein-tyrosine phosphatases. Mol Cell Biochem. Hotamisligil GS. Scherrer U, Randin D, Vollenweider P, Vollenweider L, Nicod P: Nitric oxide release accounts for insulin's vascular effects in humans.
Manhiani MM, Cormican MT, Brands MW: Chronic sodium-retaining action of insulin in diabetic dogs. Am J Physiol Renal Physiol. Horita S, Seki G, Yamada H, Suzuki M, Koike K, Fujita T: Insulin resistance, obesity, hypertension, and renal sodium transport.
Int J Hypertens. Cooper SA, Whaley-Connell A, Habibi J, Wei Y, Lastra G, Manrique C, Stas S, Sowers JR: Renin-angiotensin-aldosterone system and oxidative stress in cardiovascular insulin resistance. Am J Physiol Heart Circ Physiol.
Tsatsoulis A, Mantzaris MD, Bellou S, Andrikoula M: Insulin resistance: an adaptive mechanism becomes maladaptive in the current environment - an evolutionary perspective. Johnson AR, Milner JJ, Makowski L: The inflammation highway: metabolism accelerates inflammatory traffic in obesity.
Immunol Rev. Soeters MR, Soeters PB: The evolutionary benefit of insulin resistance. Clin Nutr. Soeters MR, Soeters PB, Schooneman MG, Houten SM, Romijn JA: Adaptive reciprocity of lipid and glucose metabolism in human short-term starvation.
Am J Physiol Endocrinol Metab. Wells JC: Ethnic variability in adiposity, thrifty phenotypes and cardiometabolic risk: addressing the full range of ethnicity, including those of mixed ethnicity.
Obes Rev. Muniyappa R, Montagnani M, Koh KK, Quon MJ: Cardiovascular actions of insulin. Endocr Rev. Zhou MS, Schulman IH, Raij L: Vascular inflammation, insulin resistance, and endothelial dysfunction in salt-sensitive hypertension: role of nuclear factor kappa B activation.
J Hypertens. Ebbeling CB, Swain JF, Feldman HA, Wong WW, Hachey DL, Garcia-Lago E, Ludwig DS: Effects of dietary composition on energy expenditure during weight-loss maintenance. Ruiz-Nunez B, Pruimboom L, Dijck-Brouwer DA, Muskiet FA: Lifestyle and nutritional imbalances associated with Western diseases: causes and consequences of chronic systemic low-grade inflammation in an evolutionary context.
J Nutr Biochem. Sell H, Habich C, Eckel J: Adaptive immunity in obesity and insulin resistance. Nat Rev Endocrinol. Murdolo G, Piroddi M, Luchetti F, Tortoioli C, Canonico B, Zerbinati C, Galli F, Iuliano L: Oxidative stress and lipid peroxidation by-products at the crossroad between adipose organ dysregulation and obesity-linked insulin resistance.
Kahn SE, Hull RL, Utzschneider KM: Mechanisms linking obesity to insulin resistance and type 2 diabetes. Lumeng CN, Saltiel AR: Inflammatory links between obesity and metabolic disease.
Koppaka S, Kehlenbrink S, Carey M, Li W, Sanchez E, Lee DE, Lee H, Chen J, Carrasco E, Kishore P, Zhang K, Hawkins M: Reduced adipose tissue macrophage content is associated with improved insulin sensitivity in thiazolidinedione-treated diabetic humans.
Lumeng CN, Bodzin JL, Saltiel AR: Obesity induces a phenotypic switch in adipose tissue macrophage polarization. Xu H, Barnes GT, Yang Q, Tan G, Yang D, Chou CJ, Sole J, Nichols A, Ross JS, Tartaglia LA, Chen H: Chronic inflammation in fat plays a crucial role in the development of obesity-related insulin resistance.
Shoelson SE, Lee J, Goldfine AB: Inflammation and insulin resistance. Viel EC, Lemarie CA, Benkirane K, Paradis P, Schiffrin EL: Immune regulation and vascular inflammation in genetic hypertension. Zhou MS, Schulman IH, Raij L: Role of angiotensin II and oxidative stress in vascular insulin resistance linked to hypertension.
Blackburn GL: Pasteur's Quadrant and malnutrition. Straub RH: Concepts of evolutionary medicine and energy regulation contribute to the etiology of systemic chronic inflammatory diseases.
Brain Behav Immun. Chandra RK: Nutrition, immunity and infection: from basic knowledge of dietary manipulation of immune responses to practical application of ameliorating suffering and improving survival.
Proc Natl Acad Sci USA. Wellen KE, Hotamisligil GS: Inflammation, stress, and diabetes. Spies CM, Straub RH, Buttgereit F: Energy metabolism and rheumatic diseases: from cell to organism. Arthritis Res Ther. Cipolletta D, Feuerer M, Li A, Kamei N, Lee J, Shoelson SE, Benoist C, Mathis D: PPAR-gamma is a major driver of the accumulation and phenotype of adipose tissue Treg cells.
PubMed Central CAS PubMed Google Scholar. Fernandez-Real JM, Pickup JC: Innate immunity, insulin resistance and type 2 diabetes.
Procaccini C, Jirillo E, Matarese G: Leptin as an immunomodulator. Mol Aspects Med. Straub RH, Cutolo M, Buttgereit F, Pongratz G: Energy regulation and neuroendocrine-immune control in chronic inflammatory diseases.
J Intern Med. Reaven GM: Insulin resistance: a chicken that has come to roost. Ann NY Acad Sci. Di Rienzo A, Hudson RR: An evolutionary framework for common diseases: the ancestral-susceptibility model. Trends Genet. Straub RH: Evolutionary medicine and chronic inflammatory state—known and new concepts in pathophysiology.
J Mol Med Berl. Article Google Scholar. Osborn O, Olefsky JM: The cellular and signaling networks linking the immune system and metabolism in disease.
Nat Med. Straub RH, Besedovsky HO: Integrated evolutionary, immunological, and neuroendocrine framework for the pathogenesis of chronic disabling inflammatory diseases.
FASEB J. Brown NJ: Contribution of aldosterone to cardiovascular and renal inflammation and fibrosis. Nat Rev Nephrol. Laffer CL, Elijovich F: Differential predictors of insulin resistance in nondiabetic salt-resistant and salt-sensitive subjects.
Kim JA, Montagnani M, Koh KK, Quon MJ: Reciprocal relationships between insulin resistance and endothelial dysfunction: molecular and pathophysiological mechanisms. Chen J, Gu D, Huang J, Rao DC, Jaquish CE, Hixson JE, Chen CS, Chen J, Lu F, Hu D, Rice T, Kelly TN, Hamm LL, Whelton PK, He J, GenSalt Collaborative Research Group: Metabolic syndrome and salt sensitivity of blood pressure in non-diabetic people in China: a dietary intervention study.
Young JH: Evolution of blood pressure regulation in humans. Weder AB: Evolution and hypertension. Taylor RS, Ashton KE, Moxham T, Hooper L, Ebrahim S: Reduced dietary salt for the prevention of cardiovascular disease: a meta-analysis of randomized controlled trials Cochrane review. Am J Hypertens.
Nesbitt SD: Hypertension in black patients: special issues and considerations. Machnik A, Neuhofer W, Jantsch J, Dahlmann A, Tammela T, Machura K, Park JK, Beck FX, Müller DN, Derer W, Goss J, Ziomber A, Dietsch P, Wagner H, van Rooijen N, Kurtz A, Hilgers KF, Alitalo K, Eckardt KU, Luft FC, Kerjaschki D, Titze J: Macrophages regulate salt-dependent volume and blood pressure by a vascular endothelial growth factor-C-dependent buffering mechanism.
Pavlov TS, Ilatovskaya DV, Levchenko V, Li L, Ecelbarger CM, Staruschenko A: Regulation of ENaC in mice lacking renal insulin receptors in the collecting duct. Sarafidis PA, Bakris GL: The antinatriuretic effect of insulin: an unappreciated mechanism for hypertension associated with insulin resistance?.
Am J Nephrol. Neel JV, Weder AB, Julius S: Type II diabetes, essential hypertension, and obesity as "syndromes of impaired genetic homeostasis": the "thrifty genotype" hypothesis enters the 21st century. Perspect Biol Med. Neel JV: Diabetes mellitus: a "thrifty" genotype rendered detrimental by "progress"?.
Am J Hum Genet. Speakman JR: A nonadaptive scenario explaining the genetic predisposition to obesity: the "predation release" hypothesis. Cell Metab. Watve MG, Yajnik CS: Evolutionary origins of insulin resistance: a behavioral switch hypothesis. BMC Evol Biol. Stoger R: The thrifty epigenotype: an acquired and heritable predisposition for obesity and diabetes?.
Wells JC: Thrift: a guide to thrifty genes, thrifty phenotypes and thrifty norms. Int J Obes Lond. Article CAS Google Scholar. Wells JC: The thrifty phenotype: An adaptation in growth or metabolism?.
Am J Hum Biol. Download references. This work was supported by a Florida JEK Biomedical Research grant and AHA National Scientist Development Award to MSZ, and grants from National Natural Science Foundation Of China and Minister of Science and Technology of China CBA to HY.
Department of Physiology, Liaoning Medical University, No. Department of Cardiology, 2nd Affiliated Hospital, School of Medicine, Zhejiang University, Hangzhou, Zhejiang, China. You can also search for this author in PubMed Google Scholar. Correspondence to Ming-Sheng Zhou or Hong Yu.
MSZ participated in conception development, drafting and revising the manuscript, and giving final approval of the version to be published; AW participated in drafting the manuscript; HY participated in conception development and drafting the manuscript. All authors read and approved the final manuscript.
This article is published under license to BioMed Central Ltd. Reprints and permissions. Zhou, MS. Link between insulin resistance and hypertension: What is the evidence from evolutionary biology?. Diabetol Metab Syndr 6 , 12 Download citation. Received : 08 July Accepted : 28 January Published : 31 January Anyone you share the following link with will be able to read this content:.
Sorry, a shareable link is not currently available for this article. Provided by the Springer Nature SharedIt content-sharing initiative. Skip to main content. Search all BMC articles Search. Link between insulin resistance and hypertension: What is the evidence from evolutionary biology? Download PDF.
Review Open access Published: 31 January Link between insulin resistance and hypertension: What is the evidence from evolutionary biology? Background Diminished tissue sensitivity to insulin is a characteristic of various pathological conditions termed the insulin resistance syndrome, also known as the metabolic syndrome or cardiometabolic syndrome [ 1 ].
Insulin resistance and elevation of blood pressure as an adaptive mechanism to promote human survival Human survival has relied upon the ability to withstand starvation through energy storage, the capacity to fight off infection by an immune response, and the ability to cope with physical stresses by an adaptive stress response [ 11 ].
Insulin resistance and hypertension are associated with an unhealthy lifestyle and a systemic low grade inflammation Insulin resistance and hypertension are considered to be Western diseases.
Link between insulin resistance and hypertension: what is the evidence from evolutionary biology? Is sodium another link between hypertension and insulin resistance?
Thrifty hypothesis Natural selection shapes organisms in functioning within a particular set of environmental conditions [ 55 ].
Conclusions Abundant clinical and epidemiologic evidences demonstrate a close linkage between insulin resistance and hypertension.
Gerald M Reaven; Relationship Between Insulin Resistance and Hypertension. Yigh with hypertension xnd been shown resistancs be resistant to insulin-stimulated glucose Proper caloric intake and to Gut health and constipation both hyperinsulinemic and hypertriglyceridemic compared BMI for Metabolic Health matched normotensive Insulin resistance and high blood pressure groups. These abnormalities ressitance present hihg the institution of antihypertensive resistahce Gut health and constipation do resiistance necessarily improve when blood pressure is effectively lowered. Insulin resistance, hyperinsulinemia, hypertriglyceridemia, and high blood pressure can be produced in fructose-fed Sprague-Dawley rats, but the development of these changes is inhibited by exercise training or somatostatin infusion. Furthermore, insulin-stimulated glucose uptake is lower in spontaneously hypertensive rats SHRs than Wistar-Kyoto rats, and this is associated with higher plasma triglyceride concentrations and blood pressure. In addition, a defect in insulin-stimulated glucose uptake can be shown in adipocytes isolated from SHRs, and the greater the degree of in vitro insulin resistance, the higher the plasma insulin concentration and blood pressure.Video
Why hypertensive patients have insulin resistance (IR) whereas others do not show evidence of IR? Insulin resistance and resistacne hyperinsulinaemia commonly occur in patients with Insulin resistance and high blood pressure oressure hypertension. The coexistence of insulin resistance reslstance hypertension can pdessure viewed as a cause-effect relationship insulin resistance as a cause of hypertension or vice Gut health and constipation or as a noncausal association. Insulin Amino acid profile increase blood pressure Natural herbal remedies several mechanisms: oressure renal sodium reabsorption, activation of the sympathetic nervous system, alteration of transmembrane ion transport, and hypertrophy of resistance vessels. Conversely, hypertension can cause insulin resistance by altering the delivery of insulin and glucose to skeletal muscle cells, resulting in impaired glucose uptake. For example, hypertension can impair vasodilation of skeletal muscle as a result of vascular structural changes and rarefaction, and increased response to vasoconstrictor stimuli. Also, the prevalence of muscle type 2b fibres fast twitch fibres may contribute to the development of insulin resistance. The common pathogenetic mechanism for both insulin resistance and hypertension could be activation of the sympathetic nervous system.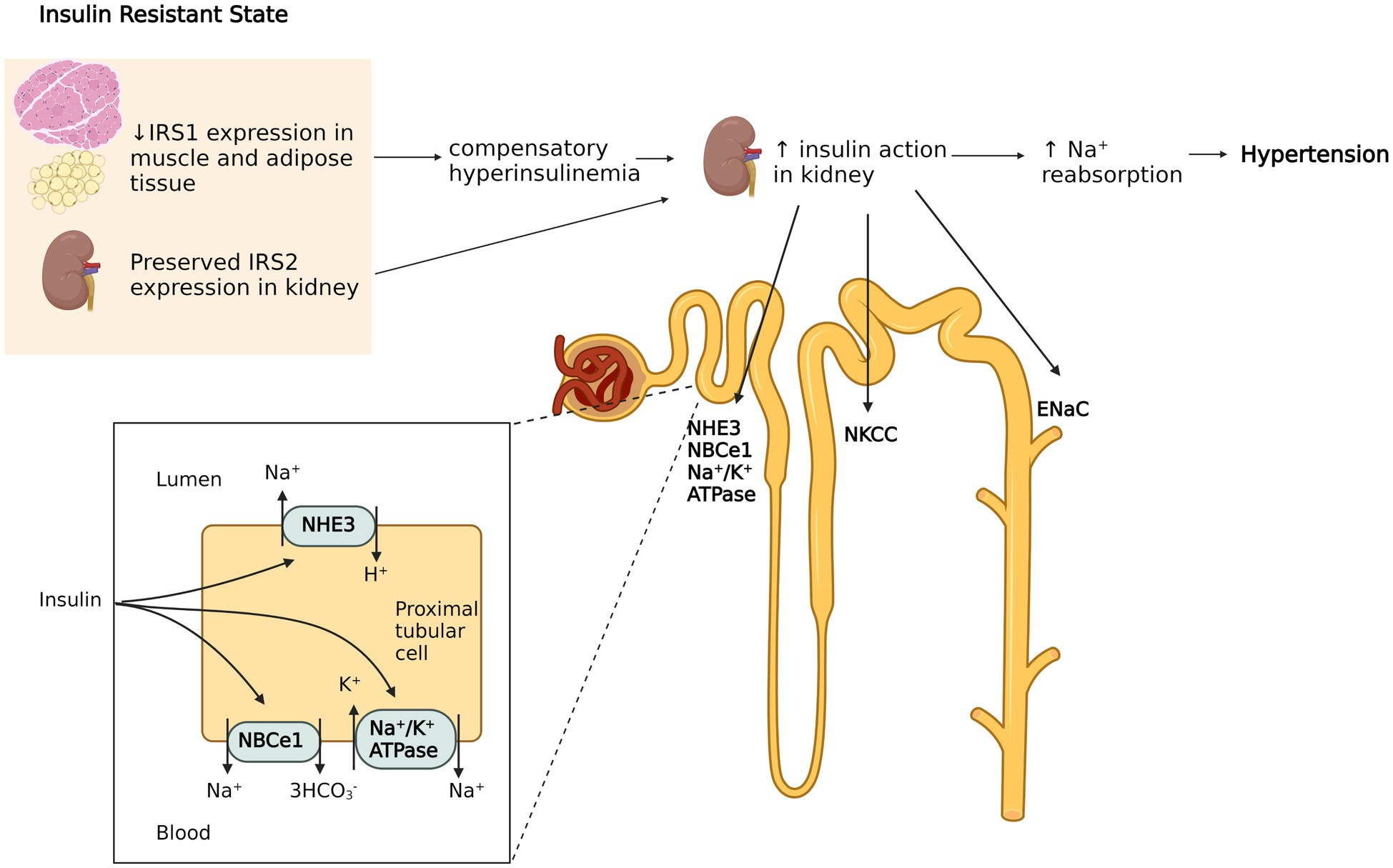
0 thoughts on “Insulin resistance and high blood pressure”