Amino acid synthesis pathway -
Injection volume was set to 1 μL for all analyses 42 min total run time per injection. MS analyses were carried out by coupling the LC system to a Thermo Q Exactive HF mass spectrometer operating in heated electrospray ionization mode HESI.
Spray voltage for both positive and negative modes was 3. Tandem MS spectra for both positive and negative mode used a resolution of 15,, AGC target of 1e5, maximum IT of 50ms, isolation window of 0. The minimum AGC target was 1e4 with an intensity threshold of 2e5.
All data were acquired in profile mode. All valine data were processed using Thermo XCalibur Qualbrowser for manual inspection and annotation of the resulting spectra and peak heights referring to authentic valine standards and labeled internal standards as described.
QIAshredder homogenizer columns Qiagen, were used to disrupt the cell lysates. Libraries were prepared using the NEBNext Ultra RNA Library Prep Kit for Illumina New England Biolabs, E , and sequenced on a NextSeq single-end 75 cycles high output with v2.
Differential gene enrichment analysis was performed with in R with DESeq2 and GO enrichment performed and visualized with clusterProfiler against the org. db database, with further visualization with the pathview, GoSemSim, eulerr packages. Target plasmid was maintained in and purified from NEB beta electrocompetent E.
coli New England Biolabs, CK. Lentivirus was packaged by plating 4×10 6 HEKT cells on 10 cm 2 and incubating cells overnight at 37°C. Cells were transfected with a plasmid mix consisting of 3. Transfected HEKT cells were incubated for 48 hr, before medium was collected, and centrifuged at ×g for 5 mins.
The resulting supernatant was filtered using a 0. The packaged virus was applied to cells for 24 hr before the medium was exchanged for fresh medium. For RNA extraction, QIAshredder homogenizer columns Qiagen, were used to disrupt the cell lysates. cDNA was generated from RNA using Invitrogen SuperScript IV Reverse Transcriptase Invitrogen, and oligo dT primers.
Each qPCR reaction was performed using SYBR Green Master I Roche, on a Light Cycler Roche, using the recommended cycling conditions.
Primers were designed to amplify amplicons — bp in size. Sequencing data generated for this study is deposited in the NCBI SRA at accession number PRJNA Source data files have been provided for Figure 1 - figure supplement 1, Figure 1 - figure supplement 2D, Figure 2, Figure 2 - figure supplement 3, Figure 2 - figure supplement 4B, Figure 2 - figure supplement 5, Figure 2 - figure supplement 6, Figure 3, and Figure 3 - figure supplement 1, Figure 4, Figure 4 - figure supplement 1, Figure 5, and Figure 5 - figure supplement 1.
Our editorial process produces two outputs: i public reviews designed to be posted alongside the preprint for the benefit of readers; ii feedback on the manuscript for the authors, including requests for revisions, shown below.
We also include an acceptance summary that explains what the editors found interesting or important about the work. Thank you for submitting your article "Resurrecting essential amino acid biosynthesis in a mammalian cell" for consideration by eLife. Your article has been reviewed by 3 peer reviewers, including Ivan Topisirovic as Reviewing Editor and Reviewer 1, and the evaluation has been overseen by Philip Cole as the Senior Editor.
The following individual involved in review of your submission has agreed to reveal their identity: Ran Kafri Reviewer 3. The reviewers have discussed their reviews with one another, and the Reviewing Editor has drafted this to help you prepare a revised submission.
Based on this, it was thought that more evidence is required to demonstrate that the introduction of valine biosynthetic pathway into CHO cells results in sustained proliferation and survival in the absence of valine supplementation.
Accordingly, it was deemed that the authors should monitor long-term ability of engineered CHO cells to sustain valine production and proliferate in valine-free media. To this end, monitoring flux via valine biosynthetic and degradation pathways, transcriptome and mTOR signaling at early and late time points was thought to be warranted.
These include lack of clarity pertinent to the rationale behind using "conditioned-medium" in the experiments. Moreover, potential utilization of other sources of valine e.
It was appreciated that the latter cells survive in valine-free media, but it seems that their proliferation is significantly lower than in valine containing media. Moreover, it seems that after 6 passages only a fraction of the detected valine is synthesized de novo.
Would this fraction further decrease in subsequent passages? Related to this, it is not clear what is the efficiency of valine biosynthesis in CHO cells vs. a prototrophic organism.
Perhaps comparing the rates of valine synthesis in cell free extracts of CHO cells vs. those derived from a prototrophic organism may be helpful to address this. This in particular relates to amino-acid sensing pathways e.
Were the enzymes mislocalized? Are there other regulatory factors involved? Moreover, considering that the overarching tenet is that metazoans lost the ability to produce essential amino acids due to energetic restraints, it may be worthwhile noting that culturing conditions and potential differences in energy resources may impact on functionalization of essential amino acid biosynthetic pathways.
The results put forth in this manuscript suggest the authors were marginally successful in introducing a valine biosynthetic pathway into CHO cells, but fall short of demonstrating a robust, self-sustaining engineered cell line under reasonable culture conditions.
This milestone should be met prior to final acceptance at eLife. Additionally, the following revisions should be carried out prior to acceptance.
The authors should identify the timepoint at which pCTRL cells are no longer viable in dropout medium. The authors should then compare transcriptional profiles of the pMTIV cells at that timepoint to the that of pMTIV cells harvested at 4hr and 48hr. Doing so may help identify key bottlenecks in the pathway.
If a bottleneck can be identified, authors should attempt to make the pathway more efficient, either by modifying expression strategy of that enzyme or testing homologs from other hosts. The pathway should be optimized until the major revision 1 above is achieved.
For clarity, these sections should be de-emphasized in writing and figures for clarity. The task that Wang et al. We thank the reviewers for this feedback. We understand the core issue to be the reduction in doubling time shown for later time points in Figure 2F and the suggestion that this represents a time-dependent lag in growth rate due to cumulative insufficient valine production.
In response to this feedback, we set out to attain a consistent doubling time in the valine-free condition. Importantly, this dihydroxy-acid dehydratase overexpressing cell line was passaged 10 times in the absence of valine with a consistent average doubling time of 3.
Doubling time remained consistent across the 39 days of culture and no medium conditioning was required Figure 5.
Nonetheless, to alleviate concerns that the original prototrophic pMTIV cells were not able to sustain proliferation long-term in the absence of valine, we have also added additional evidence indicating that these cells retained valine prototrophy long-term:.
Given the rapid death phenotype experienced by pCtrl cells, continued survival of pMTIV cells at late passages should instead be considered an indicator of sustained prototrophy. Late time point transcriptomic data Figure 3 —figure supplement 5 for pMTIV cells demonstrating partial rescue of nutritional starvation at day 29 in conditioned valine-free FK medium.
We thank the reviewers for these comments. We have added a figure highlighting mTOR signaling differences in pMTIV and pCtrl cells at 48 h valine starvation, even though no clear signatures of mTOR activation could be detected Figure 3 —figure supplement 4.
We have also added a new supplemental figure showing transcriptomic analysis of cells grown long-term 5 passages, 29 days in conditioned valine-free FK medium Figure 3 —figure supplement 5. Additionally, we were able to gain insight into flux through the pathway with 13 C-tracing.
No signal could be detected for pyruvate, 2-acetolactate or 2-oxoisovalerate; however we were able to specifically detect pathway intermediate 2,3-dihydroxy-isoverate and have added a panel to reflect this Figure 3 —figure supplement 1F. It was unclear whether the detected 2,3-dihydroxy-isoverate represented a true pathway bottleneck.
In order to test whether this was the case, we introduced extra copies of the downstream ilvD gene encoding the dihydroxy-acid dehydratase enzyme, by lentiviral transduction.
We apologize for the lack of clarity surrounding the use of conditioned medium and thank the reviewers for bringing this to our attention. We have added a panel demonstrating the utility of using conditioned medium in culturing pMTIV cells in the absence of valine Figure 2 —figure supplement 5B.
When culturing prototrophic cells in valine-free medium conditions, extracellular valine concentrations will be minimal, forcing cells to secrete valine until the appropriate equilibrium has been met.
Examples supporting this rationale can be found in the literature. For example, in a publication by Eagle and Piez 1 it was demonstrated that there is a population-dependent requirement of cultured cells for metabolites that are otherwise considered non-essential.
For instance, serine was required for growth when cells were cultured at low cell densities. Figure 2 —figure supplement 5B further supports this explanation by illustrating that the positive effect from medium conditioning cannot be recapitulated if the medium is conditioned with pCtrl cells, which excludes the possibility of cell debris or other effects from medium conditioning conferring the positive benefit.
It would therefore indicate that the benefit to cells that is derived from pMTIV medium conditioning is likely specifically caused by the valine synthesized and secreted by these cells.
The serum was analyzed for the presence of 15 amino acids including valine, which was found to be present at 9. Regarding autophagy, if such an effect would significantly alter the outcome of cells, this would not be specific to our engineered cells and any rescue effects thereof should be apparent for pCtrl cells as well, which was shown not to be the case Figure 2C, Figure 2E, Figure 2 —figure supplement 5.
Given the success with valine, we feel it appropriate to outline these results on their own terms. However, we agree that it would be beneficial to additionally discuss other efforts.
We initially began our experimentation by designing an all-in-one construct that would introduce a isoleucine and valine biosynthesis using a shared 4-gene pathway b threonine biosynthesis by driving a typically degradative enzyme in reverse, and c rescue of methionine auxotrophy by bridging a gap in the sulfur shuttle.
The all-in-one format using 2A ribosome-skipping peptide sequences served to free up the limited number of available mammalian regulatory elements for potential addition of other pathway functionalities as well as to minimize the number of genes introduced and by extension the cost of DNA synthesis.
In particular, the gene choices made in the attempts to achieve b and c were optimistic and made in the interest of optimizing pathway number per DNA length. While the valine pathway in theory is able to conduct isoleucine biosynthesis activity as well, the choice of an E.
This may be necessary for meaningful isoleucine biosynthetic functionality but in addition, isoleucine biosynthesis additionally requires the presence of 2-oxobutanoate, which is not as involved in core metabolism as pyruvate and therefore is presumably found at much lower concentrations in cells.
We have added a panel Figure 4 —figure supplement 1B demonstrating increased proliferative ability of pMTIV cells in valine-free RPMI medium at a reduced 0.
In the case of threonine, we attempted to opportunistically take advantage of the bidirectionality of a typically degradative enzyme, ltaE.
However, this failed to rescue threonine auxotrophy, presumably because the mammalian metabolic equilibrium did not favor the reverse enzymatic reaction as intended.
In the case of methionine, rescue of biosynthesis was attempted by allowing for interconversion of cystathionine and homocysteine. Methionine is synthesized in mammalian cells from homocysteine, and we reasoned that increasing levels of cystathionine by introduction of E.
coli -derived metC would increase levels of homocysteine, which might increase cell viability in methionine-free conditions.
However, cystathionine biosynthesis in E. coli and mammalian cells are divergent processes requiring different starting substrates. Whereas E. coli synthesizes cystathionine from cysteine and succinyl-homoserine, mammalian cells synthesize cystathionine from serine and homocysteine.
Introducing metC into a mammalian metabolic context therefore bridges a gap that is incompatible with the evolutionary developments of the past hundreds of millions of years, resulting in a circular pathway unlikely to produce significant quantities of methionine, which was confirmed empirically in our functional assay.
We would like to highlight to the reviewers that additional work is ongoing to rescue yet other essential amino acids, as well as our call for a wider community focus on such projects.
We would like to clarify that the metabolomics data presented in the manuscript describes a separate experiment from the long-term culture experiments, and were collected after 3 passages or 12 days in unconditioned valine-free RPMI medium containing 13 C-glucose and 13 C-sodium pyruvate Figure 3 —figure supplement 1A.
To measure valine biosynthesis past the 3 rd passage as suggested, we set out to perform an additional metabolomics analysis looking at 13 C-valine levels — this time over a longer time period. In this time course, 13 C-glucose replaced its 12 C counterpart in the valine-free RPMI medium formulation as before; however the spiked in sodium pyruvate was not 13 C-labeled in this follow-up experiment due to limited reagent availability during the COVID pandemic.
This is important to note as it follows that the expected 13 C-labeling outcome is different. This is in contrast to the original experiment in which only 13 C sources of glucose and pyruvate were spiked in.
In anticipation that cells might not perform well in unconditioned medium and in the new RPMI context, we therefore attempted to take measures to lose fewer cells to the harsh effects of passaging by culturing cells on plates coated with 0.
While it in theory was possible that cells were consuming valine derived from the 0. Furthermore, we later cultured cells long-term in unconditioned valine-free RPMI on plates not coated with 0. In pMTIV cells, 13 C-valine content was lower than 12 C-valine content on days 14 and 24 while the opposite was true on days 2, 4, 12, and 18, demonstrating that the 13 C content of the cells was not on a downward trend but rather fluctuated up and down.
This may reflect an inability to adequately respond to valine demands given inefficient flux through the pathway. We thank the reviewer for these insights. We address this point above in our response to Essential Revision 4. We agree with the reviewer on this point and have already begun efforts to test our pathways in other cell lines, such as HEK cells, but we believe these data are too preliminary to be included at this time, and are beyond the scope of this contribution.
We undertook a painstaking and extensive effort to demonstrate robust and self-sustaining valine-free growth over 39 days. This was achieved by increasing ilvD encoding a dihydroxy-acid dehydratase enzyme copy number in response to detecting a potential pathway bottleneck in 2,3-dihydroxy-isovalerate.
By increasing the efficiency of the final step in the introduced biosynthetic pathway, doubling time was reduced to a relatively consistent 3. o It is possible residual valine from complete medium may help pCTRL and pMTIV cells survive at early timepoints.
While it was possible to include an 8 day timepoint for collecting transcriptomic pMTIV samples we originally did not do so as there is no suitable control for analyzing such samples. Nonetheless, we had previously collected RNA in duplicate samples from a late time point and as such have included transcriptomic data in a new figure for samples cultured in conditioned valine-free FK medium over 29 days or 5 passages i.
well past the point of pCtrl inviability Figure 3 —figure supplement 5. Evidently, these cells more closely resemble healthy cells cultured in complete medium than do pCtrl cells cultured in valine-free FK for just 48 h.
This excellent reviewer suggestion led to an important new finding — the specific accumulation of an intermediate suggesting a pathway bottleneck.
We explored the presence of pathway intermediates in our original 13 C-tracing experiment. The only pathway intermediate detected by metabolomics analysis was 2,3-dihydroxy-isovalerate, suggesting potential bottlenecking at this step.
This resulted in cells that double on average every 3. This data demonstrates long-term homeostasis and robust growth under reasonable culturing conditions. We believed it would be misleading to describe our efforts to rescue valine biosynthesis alone.
On the suggestion of Reviewer 1 above as well as the suggestion outlined in Essential Revision 4 sections have been adjusted but not removed. The potential toxicity of valine pathway intermediates or perhaps toxic products from non-specific enzymatic activity is certainly an interesting question, given the introduction of a pathway sourced from a distantly removed species.
However, we saw no signs of metabolic stress in cells harboring the pathway when grown on complete media, either by growth rate Figure 2D or in the transcriptomic data Figure 3D, Figure 3 —figure supplement 2.
The introduction of the pathway therefore does not appear to be a significant stressor to the cells. However, it remains to be seen if this will true for all essential amino acids, particularly as we look to introduce the more complex pathways.
We thank the reviewer for the vote of confidence, and share their excitement for the insights this work might enable. Eagle, H. and Piez, K. The population-dependent requirement by cultured mammalian cells for metabolites which they can synthesize. Journal of Experimental Medicine , 29—43 Barak Z, Chipman D M, and Gollop N.
Physiological implications of the specificity of acetohydroxy acid synthase isozymes of enteric bacteria. Journal of Bacteriology , — Mitchell Leslie A.
et al. Science , eaaf Richardson Sarah M. Science , — The funders had no role in study design, data collection and interpretation, or the decision to submit the work for publication. We would like to thank the members of the Boeke and Wang labs for comments and discussion on the work and manuscript.
RMM additionally thanks personal support from Xiaoyu Weng. Defense Advanced Research Projects Agency HR HHW, JDB. National Science Foundation MCB HHW. Burroughs Wellcome Fund PATH HHW.
Irma T Hirschl Trust HHW. This article is distributed under the terms of the Creative Commons Attribution License , which permits unrestricted use and redistribution provided that the original author and source are credited. Article citation count generated by polling the highest count across the following sources: Crossref , PubMed Central , Scopus.
Apicomplexans are ubiquitous intracellular parasites of animals. These parasites use a programmed sequence of secretory events to find, invade, and then re-engineer their host cells to enable parasite growth and proliferation.
The secretory organelles micronemes and rhoptries mediate the first steps of invasion. After invasion, a second secretion programme drives host cell remodelling and occurs from dense granules.
The site s of dense granule exocytosis, however, has been unknown. In Toxoplasma gondii , small subapical annular structures that are embedded in the IMC have been observed, but the role or significance of these apical annuli to plasma membrane function has also been unknown.
Here, we determined that integral membrane proteins of the plasma membrane occur specifically at these apical annular sites, that these proteins include SNARE proteins, and that the apical annuli are sites of vesicle fusion and exocytosis. Specifically, we show that dense granules require these structures for the secretion of their cargo proteins.
When secretion is perturbed at the apical annuli, parasite growth is strongly impaired. The apical annuli, therefore, represent a second type of IMC-embedded structure to the apical complex that is specialised for protein secretion, and reveal that in Toxoplasma there is a physical separation of the processes of pre- and post-invasion secretion that mediate host-parasite interactions.
Cellular metabolism plays an essential role in the regrowth and regeneration of a neuron following physical injury. Yet, our knowledge of the specific metabolic pathways that are beneficial to neuron regeneration remains sparse.
Previously, we have shown that modulation of O-linked β-N-acetylglucosamine O-GlcNAc signaling, a ubiquitous post-translational modification that acts as a cellular nutrient sensor, can significantly enhance in vivo neuron regeneration.
Here, we define the specific metabolic pathway by which O-GlcNAc transferase ogt-1 loss of function mediates increased regenerative outgrowth. Performing in vivo laser axotomy and measuring subsequent regeneration of individual neurons in C.
elegans , we find that glycolysis, serine synthesis pathway SSP , one-carbon metabolism OCM , and the downstream transsulfuration metabolic pathway TSP are all essential in this process.
Testing downstream branches of this pathway, we find that enhanced regeneration is dependent only on the vitamin B12 independent shunt pathway. These results are further supported by RNA sequencing that reveals dramatic transcriptional changes by the ogt-1 mutation, in the genes involved in glycolysis, OCM, TSP, and ATP metabolism.
Strikingly, the beneficial effects of the ogt-1 mutation can be recapitulated by simple metabolic supplementation of the OCM metabolite methionine in wild-type animals. Taken together, these data unearth the metabolic pathways involved in the increased regenerative capacity of a damaged neuron in ogt-1 animals and highlight the therapeutic possibilities of OCM and its related pathways in the treatment of neuronal injury.
Mitochondrial membrane potential directly powers many critical functions of mitochondria, including ATP production, mitochondrial protein import, and metabolite transport. Its loss is a cardinal feature of aging and mitochondrial diseases, and cells closely monitor membrane potential as an indicator of mitochondrial health.
Given its central importance, it is logical that cells would modulate mitochondrial membrane potential in response to demand and environmental cues, but there has been little exploration of this question. We report that loss of the Sit4 protein phosphatase in yeast increases mitochondrial membrane potential, both by inducing the electron transport chain and the phosphate starvation response.
Indeed, a similarly elevated mitochondrial membrane potential is also elicited simply by phosphate starvation or by abrogation of the Phodependent phosphate sensing pathway.
We also demonstrate that this connection between phosphate limitation and enhancement of mitochondrial membrane potential is observed in primary and immortalized mammalian cells as well as in Drosophila.
These data suggest that mitochondrial membrane potential is subject to environmental stimuli and intracellular signaling regulation and raise the possibility for therapeutic enhancement of mitochondrial function even in defective mitochondria.
Share this article Doi. Cite this article Julie Trolle Ross M McBee Andrew Kaufman Sudarshan Pinglay Henri Berger Sergei German Liyuan Liu Michael J Shen Xinyi Guo J Andrew Martin Michael E Pacold Drew R Jones Jef D Boeke Harris H Wang Resurrecting essential amino acid biosynthesis in mammalian cells.
Full text Figures and data Side by side Abstract Editor's evaluation Main text Data availability References Decision letter Author response Article and author information Metrics. sa0 Decision letter Reviews on Sciety eLife's review process.
Figure 1 with 2 supplements see all. Download asset Open asset. Figure 2 with 6 supplements see all. Figure 2—source data 1 Raw cell count data for pMTIV valine-free and complete FK medium tests.
zip Download elifefig2-data1-v2. Figure 3 with 5 supplements see all. zip Download elifefig3-data1-v2. Figure 4 with 1 supplement see all. zip Download elifefig4-data1-v2.
Figure 5 with 1 supplement see all. zip Download elifefig5-data1-v2. Key resources table. Reagent type species or resource Designation Source or reference Identifiers Additional information Strain, strain background E. coli TransforMax EPI Lucigen CC Chemically competent Strain, strain background E.
coli NEB beta New England Biolabs CK Electrocompetent Cell line C. griseus CHO Flp-In ThermoFisher R Cell line C. griseus CHO pMTIV This paper Cell line maintained in J.
Boeke lab Cell line C. griseus CHO pCtrl This paper Cell line maintained in J. griseus CHO pPro This paper Cell line maintained in H. Wang lab Cell line C. griseus CHO pCtrl-mCh This paper Cell line maintained in H. The following data sets were generated.
Trolle J NCBI BioProject ID PRJNA Data from: Resurrecting essential amino acid biosynthesis in a mammalian cell. Amorim Franco TM Blanchard JS Bacterial branched-chain amino acid biosynthesis: structures, mechanisms, and drugability Biochemistry 56 — Barak Z Chipman DM Gollop N Physiological implications of the specificity of acetohydroxy acid synthase isozymes of enteric bacteria Journal of Bacteriology — Black JB Perez-Pinera P Gersbach CA Mammalian synthetic biology: engineering biological systems Annual Review of Biomedical Engineering 19 — Blau N van Spronsen FJ Levy HL Phenylketonuria Lancet — Boeke JD Church G Hessel A Kelley NJ Arkin A Cai Y Carlson R Chakravarti A Cornish VW Holt L Isaacs FJ Kuiken T Lajoie M Lessor T Lunshof J Maurano MT Mitchell LA Rine J Rosser S Sanjana NE Silver PA Valle D Wang H Way JC Yang L The genome project-write Science — Cunningham JA Liu AG Bengtson S Donoghue PCJ The origin of animals: can molecular clocks and the fossil record be reconciled?
BioEssays 39 :1— Dancis J Levitz M Westall RG Maple syrup urine disease: branched-chain keto-aciduria Pediatrics 25 — Eagle H Piez K The population-dependent requirement by cultured mammalian cells for metabolites which they can synthesize The Journal of Experimental Medicine — Fischer S Handrick R Otte K The art of CHO cell engineering: a comprehensive retrospect and future perspectives Biotechnology Advances 33 — Fredens J Wang K de la Torre D Funke LFH Robertson WE Christova Y Chia T Schmied WH Dunkelmann DL Beránek V Uttamapinant C Llamazares AG Elliott TS Chin JW Total synthesis of Escherichia coli with a recoded genome Nature — Gilbert LA Horlbeck MA Adamson B Villalta JE Chen Y Whitehead EH Guimaraes C Panning B Ploegh HL Bassik MC Qi LS Kampmann M Weissman JS Genome-Scale CRISPR-mediated control of gene repression and activation Cell — Guedes RLM Prosdocimi F Fernandes GR Moura LK Ribeiro HAL Ortega JM Amino acids biosynthesis and nitrogen assimilation pathways: a great genomic deletion during eukaryotes evolution BMC Genomics 12 Suppl 4 :S2.
Hefzi H Ang KS Hanscho M Bordbar A Ruckerbauer D Lakshmanan M Orellana CA Baycin-Hizal D Huang Y Ley D Martinez VS Kyriakopoulos S Jiménez NE Zielinski DC Quek L-E Wulff T Arnsdorf J Li S Lee JS Paglia G Loira N Spahn PN Pedersen LE Gutierrez JM King ZA Lund AM Nagarajan H Thomas A Abdel-Haleem AM Zanghellini J Kildegaard HF Voldborg BG Gerdtzen ZP Betenbaugh MJ Palsson BO Andersen MR Nielsen LK Borth N Lee D-Y Lewis NE A consensus genome-scale reconstruction of Chinese hamster ovary cell metabolism Cell Systems 3 — Heng BC Aubel D Fussenegger M Prosthetic gene networks as an alternative to standard pharmacotherapies for metabolic disorders Current Opinion in Biotechnology 35 — The is deacetylated and enters the urea cycle.
Here we present just the synthesis of lysine from aspartate and pyruvate using the diaminopimelic acid DAP pathway. Fundamentals of Biochemistry Vol. II - Bioenergetics and Metabolism. jpg" ]. Search site Search Search. Go back to previous article. Sign in. II - Bioenergetics and Metabolism Biosynthesis of Amino Acids, Nucleotides, and Related Molecules Introduction By the time many students get to the study of amino acid biosynthesis, they have seen so many pathways that learning new pathways for the amino acids seems daunting, even though they can be clustered into subpathways.
From Pyruvate: Ala, Val, Leu, Ile Ala can easily be synthesized from the alpha-keto acid pyruvate by a transamination reaction, so we will focus our attention on the others, the branched-chain nonpolar amino acids Val, Leu, and Ile.
TCA Intermediates From α-ketogluatarate: Glu, Gln, Pro, Arg Since amino acid metabolism is so complex, it's important to constantly review past learning. From oxalacetate: Asp, Asn, Met, Thr, Lys OAA to Aspartatic Acid This is a simple transamination.
Aspartic Acid to Lysine There are two pathways. The diaminopimelic acid DAP pathway uses aspartate and pyruvate and forms diaminopimelic acid as an intermediate. It's found in bacteria, some fungi, and archaea and in plants.
If ptahway Amino acid synthesis pathway acidd message, it syntbesis we're having trouble loading external resources on our website. org are unblocked. To log in and use all the features of Khan Academy, please enable JavaScript in your browser. Get AI Tutoring NEW. Search for courses, skills, and videos. About About this video Transcript.Synthhesis acids are the structural units that make up proteins. Amino acid synthesis pathway snythesis together to form short polymer chains called acie or longer synthwsis called either polypeptides or proteins. These Fertility diet plan are Amino acid synthesis pathway and unbranched, syjthesis each amino acid within the chain attached to two Amino acid synthesis pathway amino Synthdsis.
The process of making proteins is called translation and involves Amino acid synthesis pathway step-by-step addition of Amino acid synthesis pathway acids to a growing protein chain by a ribozyme Gut health is called a ribosome. Amion amino acids synthesix naturally incorporated into polypeptides Amino acid synthesis pathway are pathwaay proteinogenic or Dynthesis amino syjthesis.
Of these, synthexis are encoded ppathway the universal genetic code. The remaining Amino acid synthesis pathway, selenocysteine and patuway, are ackd into proteins by unique sythesis mechanisms.
Selenocysteine synthewis incorporated when pathwwy mRNA being translated includes a SECIS element, which causes the UGA Ammino to acix selenocysteine instead of a stop Amino acid synthesis pathway.
Pyrrolysine is Amono by some adid archaea in enzymes that they use synthesls produce methane. It is coded with the codon UAG, which Green tea extract and brain function normally pathwayy stop codon in other organisms.
Synhhesis abbreviated as Pyl patheay Amino acid synthesis pathway is a naturally occurring amino acid similar to lysine, but with an added acidd ring Amibo to the end of the lysine side chain.
Produced pathwau a pzthway tRNA and aminoacyl Green tea for relaxation synthetase, it synthfsis part of pathwxy unusual genetic code in these Aminno.
It is considered patuway 22 nd proteinogenic amino syntbesis. This Wcid codon is followed by Amino acid synthesis pathway Synthexis downstream sequence. Organisms vary in their ability to synthesize the 20 common amino acids. Most bacteria and plants can synthesize all Some simple parasites, such as the bacteria Mycoplasma pneumoniaelack all amino acid synthesis and take their amino acids directly from their hosts.
All amino acids are synthesized from intermediates in glycolysis, the citric acid cycle, or the pentose phosphate pathway. Nitrogen is provided by glutamate and glutamine. Amino acid synthesis depends on the formation of the appropriate alpha-keto acid, which is then transaminated to form an amino acid.
Amino acids are made into proteins by being joined together in a chain by peptide bonds. Each different protein has a unique sequence of amino acid residues: this is its primary structure.
Just as the letters of the alphabet can be combined to form an almost endless variety of words, amino acids can be linked in varying sequences to form a huge variety of proteins.
Proteins are made from amino acids that have been activated by attachment to a transfer RNA molecule through an ester bond. Aside from the 22 standard amino acids, there are many other amino acids that are called non-proteinogenic or non-standard.
Those either are not found in proteins for example carnitine, GABA or are not produced directly and in isolation by standard cellular machinery for example, hydroxyproline and selenomethionine. Non-standard amino acids that are found in proteins are formed by post-translational modification, which is modification after translation during protein synthesis.
These modifications are often essential for the function or regulation of a protein. For example, the carboxylation of glutamate allows for better binding of calcium cations.
The hydroxylation of proline is critical for maintaining connective tissues. Another example is the formation of hypusine in the translation initiation factor EIF5A, through modification of a lysine residue.
Such modifications can also determine the localization of the protein, e. Some nonstandard amino acids are not found in proteins. Examples include lanthionine, 2-aminoisobutyric acid, dehydroalanine, and the neurotransmitter gamma-aminobutyric acid.
Nonstandard amino acids often occur as intermediates in the metabolic pathways for standard amino acids — for example, ornithine and citrulline occur in the urea cycle, part of amino acid catabolism. Search site Search Search. Go back to previous article. Sign in. Learning Objectives Recognize the factors involved in amino acid synthesis.
Key Points All amino acids are synthesized from intermediates in glycolysis, the citric acid cycle, or the pentose phosphate pathway.
Of the 22 amino acids naturally incorporated into proteins, 20 are encoded by the universal genetic code and the remaining two, selenocysteine and pyrrolysine, are incorporated into proteins by unique synthetic mechanisms. Key Terms pyrrolysine : An amino acid found in methanogenic bacteria. selenocysteine : A naturally-occurring amino acid, present in several enzymes, whose structure is that of cysteine but with the sulfur atom replaced by one of selenium.
genetic code : The set of rules by which the sequence of bases in DNA are translated into the amino acid sequence of proteins.
: Amino acid synthesis pathwayAmino acid synthesis - Wikipedia | Another important syntheeis evolution mechanism is horizontal gene AmihoAmino acid synthesis pathway allows the rapid acquisition of fully functional pathwah and pathways. Amino acid synthesis pathway thank the Cholesterol level and exercise recommendations for these insights. In Wendisch VF ed. Departamento de Ciencias Naturales, Universidad Autonoma Metropolitana © Nature Education. They join together to form short polymer chains called peptides or longer chains called either polypeptides or proteins. While it was possible to include an 8 day timepoint for collecting transcriptomic pMTIV samples we originally did not do so as there is no suitable control for analyzing such samples. CHO Flp-In cells ThermoFisher, R were used in all experiments. |
Alpha amino acid synthesis (video) | Khan Academy | coli synthesizes cystathionine from cysteine and succinyl-homoserine, mammalian cells synthesize cystathionine from serine and homocysteine. Mind Read. His4 then catalyzes the formation of phosphoribosylformiminoAICAR-phosphate, which is then converted to phosphoribulosylformimino-AICAR-P by the His6 gene product. Payne SH Loomis WF Retention and loss of amino acid biosynthetic pathways based on analysis of whole-genome sequences Eukaryotic Cell 5 — Phosphoglycerate dehydrogenase is regulated by the concentration of serine in the cell. E Growth curve of CHO-K1 cells with pCtrl or pMTIV in valine-free FK medium Figure 2—source data 1. |
Resurrecting essential amino acid biosynthesis in mammalian cells | eLife | These 11 are called the non-essential amino acids. Most amino acids are synthesized from α- ketoacids , and later transaminated from another amino acid, usually glutamate. The enzyme involved in this reaction is an aminotransferase. Glutamate itself is formed by amination of α-ketoglutarate :. The α-ketoglutarate family of amino acid synthesis synthesis of glutamate, glutamine, proline and arginine begins with α-ketoglutarate, an intermediate in the Citric Acid Cycle. The concentration of α-ketoglutarate is dependent on the activity and metabolism within the cell along with the regulation of enzymatic activity. coli citrate synthase, the enzyme involved in the condensation reaction initiating the Citric Acid Cycle is strongly inhibited by α-ketoglutarate feedback inhibition and can be inhibited by DPNH as well high concentrations of ATP. The regulation of the synthesis of glutamate from α-ketoglutarate is subject to regulatory control of the Citric Acid Cycle as well as mass action dependent on the concentrations of reactants involved due to the reversible nature of the transamination and glutamate dehydrogenase reactions. The conversion of glutamate to glutamine is regulated by glutamine synthetase GS and is a key step in nitrogen metabolism. Repression and depression due to nitrogen levels; 2. Activation and inactivation due to enzymatic forms taut and relaxed ; 3. Cumulative feedback inhibition through end product metabolites; and 4. Alterations of the enzyme due to adenylation and deadenylation. The taut form of GS is fully active but, the removal of manganese converts the enzyme to the relaxed state. The specific conformational state occurs based on the binding of specific divalent cations and is also related to adenylation. Glutamine and a regulatory protein called PII act together to stimulate adenylation. The regulation of proline biosynthesis can depend on the initial controlling step through negative feedback inhibition. coli , proline allosterically inhibits Glutamate 5-kinase which catalyzes the reaction from L-glutamate to an unstable intermediate L-γ-Glutamyl phosphate. Arginine synthesis also utilizes negative feedback as well as repression through a repressor encoded by the gene argR. The gene product of argR , ArgR an aporepressor , and arginine as a corepressor affect the operon of arginine biosynthesis. The degree of repression is determined by the concentrations of the repressor protein and corepressor level. Phenylalanine , tyrosine , and tryptophan , the aromatic amino acids , arise from chorismate. Each one of these has its synthesis regulated from tyrosine, phenylalanine, and tryptophan, respectively. The rest of the enzymes in the common pathway conversion of DAHP to chorismate appear to be synthesized constitutively, except for shikimate kinase , which can be inhibited by shikimate through linear mixed-type inhibition. Tyrosine and phenylalanine are biosynthesized from prephenate , which is converted to an amino acid-specific intermediate. This process is mediated by a phenylalanine PheA or tyrosine TyrA specific chorismate mutase-prephenate dehydrogenase. PheA uses a simple dehydrogenase to convert prephenate to phenylpyruvate , while TyrA uses a NAD-dependent dehydrogenase to make 4-hydroxylphenylpyruvate. Both PheA and TyrA are feedback inhibited by their respective amino acids. Tyrosine can also be inhibited at the transcriptional level by the TyrR repressor. TyrR binds to the TyrR boxes on the operon near the promoter of the gene that it wants to repress. Tryptophan biosynthesis involves conversion of chorismate to anthranilate using anthranilate synthase. This enzyme requires either glutamine as the amino group donor or ammonia itself. Anthranilate synthase is regulated by the gene products of trpE and trpG. trpE encodes the first subunit, which binds to chorismate and moves the amino group from the donor to chorismate. trpG encodes the second subunit, which facilitates the transfer of the amino group from glutamine. Anthranilate synthase is also regulated by feedback inhibition: tryptophan is a co-repressor to the TrpR repressor. Aspartate can be converted into lysine, asparagine, methionine and threonine. Threonine also gives rise to isoleucine. As is typical in highly branched metabolic pathways, additional regulation at each branch point of the pathway. This type of regulatory scheme allows control over the total flux of the aspartate pathway in addition to the total flux of individual amino acids. The aspartate pathway uses L-aspartic acid as the precursor for the biosynthesis of one fourth of the building block amino acids. The enzyme aspartokinase , which catalyzes the phosphorylation of aspartate and initiates its conversion into other amino acids, can be broken up into 3 isozymes, AK-I, II and III. AK-I is feed-back inhibited by threonine , while AK-II and III are inhibited by lysine. As a sidenote, AK-III catalyzes the phosphorylation of aspartic acid that is the committed step in this biosynthetic pathway. Aspartate kinase becomes downregulated by the presence of threonine or lysine. Lysine is synthesized from aspartate via the diaminopimelate DAP pathway. The initial two stages of the DAP pathway are catalyzed by aspartokinase and aspartate semialdehyde dehydrogenase. These enzymes play a key role in the biosynthesis of lysine , threonine , and methionine. Transcription of aspartokinase genes is regulated by concentrations of the subsequently produced amino acids, lysine, threonine, and methionine. The higher these amino acids concentrations, the less the gene is transcribed. ThrA and LysC are also feed-back inhibited by threonine and lysine. Finally, DAP decarboxylase LysA mediates the last step of the lysine synthesis and is common for all studied bacterial species. The formation of aspartate kinase AK , which catalyzes the phosphorylation of aspartate and initiates its conversion into other amino acids, is also inhibited by both lysine and threonine , which prevents the formation of the amino acids derived from aspartate. Additionally, high lysine concentrations inhibit the activity of dihydrodipicolinate synthase DHPS. So, in addition to inhibiting the first enzyme of the aspartate families biosynthetic pathway, lysine also inhibits the activity of the first enzyme after the branch point, i. the enzyme that is specific for lysine's own synthesis. The biosynthesis of asparagine originates with aspartate using a transaminase enzyme. The enzyme asparagine synthetase produces asparagine, AMP , glutamate, and pyrophosphate from aspartate, glutamine , and ATP. In the asparagine synthetase reaction, ATP is used to activate aspartate, forming β-aspartyl-AMP. Glutamine donates an ammonium group, which reacts with β-aspartyl-AMP to form asparagine and free AMP. Two asparagine synthetases are found in bacteria. Both are referred to as the AsnC protein. They are coded for by the genes AsnA and AsnB. AsnC is autogenously regulated, which is where the product of a structural gene regulates the expression of the operon in which the genes reside. The stimulating effect of AsnC on AsnA transcription is downregulated by asparagine. However, the autoregulation of AsnC is not affected by asparagine. Biosynthesis by the transsulfuration pathway starts with aspartic acid. Relevant enzymes include aspartokinase , aspartate-semialdehyde dehydrogenase , homoserine dehydrogenase , homoserine O-transsuccinylase , cystathionine-γ-synthase , Cystathionine-β-lyase in mammals, this step is performed by homocysteine methyltransferase or betaine—homocysteine S-methyltransferase. Methionine biosynthesis is subject to tight regulation. The repressor protein MetJ, in cooperation with the corepressor protein S-adenosyl-methionine, mediates the repression of methionine's biosynthesis. The regulator MetR is required for MetE and MetH gene expression and functions as a transactivator of transcription for these genes. MetR transcriptional activity is regulated by homocystein, which is the metabolic precursor of methionine. It is also known that vitamin B12 can repress MetE gene expression, which is mediated by the MetH holoenzyme. In plants and microorganisms, threonine is synthesized from aspartic acid via α-aspartyl-semialdehyde and homoserine. Homoserine undergoes O -phosphorylation; this phosphate ester undergoes hydrolysis concomitant with relocation of the OH group. The biosynthesis of threonine is regulated via allosteric regulation of its precursor, homoserine , by structurally altering the enzyme homoserine dehydrogenase. This reaction occurs at a key branch point in the pathway, with the substrate homoserine serving as the precursor for the biosynthesis of lysine, methionine, threonin and isoleucine. High levels of threonine result in low levels of homoserine synthesis. The synthesis of aspartate kinase AK , which catalyzes the phosphorylation of aspartate and initiates its conversion into other amino acids, is feed-back inhibited by lysine , isoleucine , and threonine , which prevents the synthesis of the amino acids derived from aspartate. So, in addition to inhibiting the first enzyme of the aspartate families biosynthetic pathway, threonine also inhibits the activity of the first enzyme after the branch point, i. the enzyme that is specific for threonine's own synthesis. As is evident from the figure, glutamic acid can be made directly through the transamination of α-ketoglutarate by an ammonia donor, while glutamine can be made by the action of glutamine synthase on glutamic acid. Arginine is synthesized in the urea cycle as we have seen before. It can be made from α-ketoglutarate through the following sequential intermediates: N-acetylglutamate, N-acetylglutamate-phosphate, N-acetylglutamate-semialdehyde, N-acetylornithine to N-acetylcitruline. The is deacetylated and enters the urea cycle. Here we present just the synthesis of lysine from aspartate and pyruvate using the diaminopimelic acid DAP pathway. Fundamentals of Biochemistry Vol. II - Bioenergetics and Metabolism. jpg" ]. Search site Search Search. Go back to previous article. Nitrogen is provided by glutamate and glutamine. Amino acid synthesis depends on the formation of the appropriate alpha-keto acid, which is then transaminated to form an amino acid. Amino acids are made into proteins by being joined together in a chain by peptide bonds. Each different protein has a unique sequence of amino acid residues: this is its primary structure. Just as the letters of the alphabet can be combined to form an almost endless variety of words, amino acids can be linked in varying sequences to form a huge variety of proteins. Proteins are made from amino acids that have been activated by attachment to a transfer RNA molecule through an ester bond. Aside from the 22 standard amino acids, there are many other amino acids that are called non-proteinogenic or non-standard. Those either are not found in proteins for example carnitine, GABA or are not produced directly and in isolation by standard cellular machinery for example, hydroxyproline and selenomethionine. Non-standard amino acids that are found in proteins are formed by post-translational modification, which is modification after translation during protein synthesis. These modifications are often essential for the function or regulation of a protein. For example, the carboxylation of glutamate allows for better binding of calcium cations. The hydroxylation of proline is critical for maintaining connective tissues. Another example is the formation of hypusine in the translation initiation factor EIF5A, through modification of a lysine residue. Such modifications can also determine the localization of the protein, e. |
5.14A: Amino Acid Synthesis | Furthermore, the bottom extension of basic amino acids appears to be most divergent containing multiple pathways for lysine biosynthesis and multiple gene sets for arginine biosynthesis. Image resolution: High. Link: Normal Module. Reactions beginning with either one or two molecules of pyruvate lead to the synthesis of alanine, valine, and leucine. Feedback inhibition of final products is the main method of inhibition, and, in E. coli , the ilvEDA operon also plays a part in this regulation. Alanine is produced by the transamination of one molecule of pyruvate using two alternate steps: 1 conversion of glutamate to α-ketoglutarate using a glutamate-alanine transaminase, and 2 conversion of valine to α-ketoisovalerate via Transaminase C. Not much is known about the regulation of alanine synthesis. The only definite method is the bacterium's ability to repress Transaminase C activity by either valine or leucine see ilvEDA operon. Other than that, alanine biosynthesis does not seem to be regulated. Valine is produced by a four-enzyme pathway. It begins with the condensation of two equivalents of pyruvate catalyzed by acetohydroxy acid synthase yielding α-acetolactate. This is catalyzed by acetohydroxy isomeroreductase. The third step is the dehydration of α, β-dihydroxyisovalerate catalyzed by dihydroxy acid dehydrase. In the fourth and final step, the resulting α-ketoisovalerate undergoes transamination catalyzed either by an alanine-valine transaminase or a glutamate-valine transaminase. Valine biosynthesis is subject to feedback inhibition in the production of acetohydroxy acid synthase. The leucine synthesis pathway diverges from the valine pathway beginning with α-ketoisovalerate. α-Isopropylmalate synthase catalyzes this condensation with acetyl CoA to produce α-isopropylmalate. An isomerase converts α-isopropylmalate to β-isopropylmalate. The final step is the transamination of the α-ketoisocaproate by the action of a glutamate-leucine transaminase. Leucine, like valine, regulates the first step of its pathway by inhibiting the action of the α-Isopropylmalate synthase. The genes that encode both the dihydroxy acid dehydrase used in the creation of α-ketoisovalerate and Transaminase E, as well as other enzymes are encoded on the ilvEDA operon. This operon is bound and inactivated by valine , leucine , and isoleucine. Isoleucine is not a direct derivative of pyruvate, but is produced by the use of many of the same enzymes used to produce valine and, indirectly, leucine. When one of these amino acids is limited, the gene furthest from the amino-acid binding site of this operon can be transcribed. When a second of these amino acids is limited, the next-closest gene to the binding site can be transcribed, and so forth. The commercial production of amino acids usually relies on mutant bacteria that overproduce individual amino acids using glucose as a carbon source. Some amino acids are produced by enzymatic conversions of synthetic intermediates. Aspartic acid is produced by the addition of ammonia to fumarate using a lyase. See Template:Leucine metabolism in humans — this diagram does not include the pathway for β-leucine synthesis via leucine 2,3-aminomutase. Contents move to sidebar hide. Article Talk. Read Edit View history. Tools Tools. What links here Related changes Upload file Special pages Permanent link Page information Cite this page Get shortened URL Download QR code Wikidata item. Download as PDF Printable version. The set of biochemical processes by which amino acids are produced. For the non-biological synthesis of amino acids, see Strecker amino acid synthesis. Demand Media. Retrieved 28 July Annual Review of Microbiology. doi : PMID The physiology and biochemistry of prokaryotes 3rd ed. New York: Oxford Univ. ISBN Journal of Molecular Biology. PMC Principles of Biochemistry 3rd ed. New York: W. Lehninger, Principles of Biochemistry 3rd ed. New York: Worth Publishing. Microbial Biotechnology. ISSN The Journal of Biological Chemistry. Amino Acids. S2CID The Biosynthesis of Histidine and Its Regulation. Archived from the original on 9 December Retrieved 29 April International Journal of Biochemistry. In Wendisch VF ed. Amino acid biosynthesis: pathways, regulation, and metabolic engineering. Berlin: Springer. Annual Review of Biochemistry. Ullmann's Encyclopedia of Industrial Chemistry. Weinheim: Wiley-VCH. Gene expression. Bacterial Archaeal Eukaryotic. Transcription factor RNA polymerase Promoter. Ribosome Transfer RNA tRNA Ribosome-nascent chain complex RNC Post-translational modification. Epigenetic imprinting Transcriptional Gene regulatory network cis-regulatory element lac operon Post-transcriptional sequestration P-bodies alternative splicing microRNA Translational Post-translational reversible irreversible. François Jacob Jacques Monod. Metabolism , catabolism , anabolism. Metabolic pathway Metabolic network Primary nutritional groups. Purine metabolism Nucleotide salvage Pyrimidine metabolism Purine nucleotide cycle. Pentose phosphate pathway Fructolysis Polyol pathway Galactolysis Leloir pathway. Glycosylation N-linked O-linked. Photosynthesis Anoxygenic photosynthesis Chemosynthesis Carbon fixation DeLey-Doudoroff pathway Entner-Doudoroff pathway. Xylose metabolism Radiotrophism. Fatty acid degradation Beta oxidation Fatty acid synthesis. Steroid metabolism Sphingolipid metabolism Eicosanoid metabolism Ketosis Reverse cholesterol transport. Metal metabolism Iron metabolism Ethanol metabolism Phospagen system ATP-PCr. Metabolism : Protein metabolism , synthesis and catabolism enzymes. Get AI Tutoring NEW. Search for courses, skills, and videos. About About this video Transcript. Created by Tracy Kim Kovach. Want to join the conversation? Log in. Sort by: Top Voted. Aditya Bhattad. Posted 10 years ago. Downvote Button navigates to signup page. Flag Button navigates to signup page. Show preview Show formatting options Post answer. At biological PH 7 the amino acid ends are both charged. After the hydrolyzed step, why does the heat only remove one acid group as opposed to both? Cthulhu Mittens. Posted 9 years ago. you need the second carbonyl group to act as an electron sink. Comment Button navigates to signup page. at It is a pretty long mechanism. Basically what happens is the nitrogen in the cyanide keeps getting protonated by the acid until it wants to leave the carbon. While this is happening the carbon from the cyanide is gaining water from the acid group being deprotonated by the nitrogen until it is a carboxylic acid. Here is a link to a website that shows the steps with the mechanism. Posted a year ago. personally im into gabriel synthesis more. Do both reactions produce a racemic mixture of amino acids? yes they both do since both synthesis start with a planar molecule! Eric Dornoff. Posted 8 years ago. |
Resurrecting essential amino acid biosynthesis in mammalian cells | Comment Button navigates to signup page. at It is a pretty long mechanism. Basically what happens is the nitrogen in the cyanide keeps getting protonated by the acid until it wants to leave the carbon. While this is happening the carbon from the cyanide is gaining water from the acid group being deprotonated by the nitrogen until it is a carboxylic acid. Here is a link to a website that shows the steps with the mechanism. Posted a year ago. personally im into gabriel synthesis more. Do both reactions produce a racemic mixture of amino acids? yes they both do since both synthesis start with a planar molecule! Eric Dornoff. Posted 8 years ago. If you are synthesizing an amino acid with a more reactive R group - say glutamate or arginine - how do you prevent the R group from participating in either synthesis Strecker or Gabriel? Daniel Isaac. Posted 6 years ago. There are protecting groups available for protecting essentially every R group found on an amino acid. Such amino acid derivatives are typically used in solid phase peptide synthesis when a long peptide is desired. Posted 7 years ago. yes, because both are starting with planar molecules, sorry for responding 6 years late hope you graduated as a doctor lol. is this is all what we have to know about Gabriel synthesis for the MCAT? Aadim Npl. Posted 5 years ago. Where is n-phthalimidomalonic ester found? Is it found in food? Darren Savage. Is this a part of content category 1A? Video transcript Hey. So we're going to be talking about amino acid synthesis. And we're just going to stick with two of the main methods for synthesizing amino acids. Link: Normal Module. ID search. Reaction module Reaction modules Carboxylic acid metabolism 2-Oxocarboxylic acid chain extension RM 2-Oxocarboxylic acid chain extension by tricarboxylic acid pathway 2-Oxocarboxylic acid chain modification RM Carboxyl to amino conversion using protective N-acetyl group basic amino acid synthesis RM Carboxyl to amino conversion without using protective group RM Branched-chain addition branched-chain amino acid synthesis. In this case NAS will act to disallow the binding of CysB to its own DNA sequence. OAS is a precursor of NAS, cysteine itself can inhibit CysE which functions to create OAS. Without the necessary OAS, NAS will not be produced and cysteine will not be produced. There are two other negative regulators of cysteine. These are the molecules sulfide and thiosulfate , they act to bind to CysB and they compete with NAS for the binding of CysB. Pyruvate, the result of glycolysis , can feed into both the TCA cycle and fermentation processes. Reactions beginning with either one or two molecules of pyruvate lead to the synthesis of alanine, valine, and leucine. Feedback inhibition of final products is the main method of inhibition, and, in E. coli , the ilvEDA operon also plays a part in this regulation. Alanine is produced by the transamination of one molecule of pyruvate using two alternate steps: 1 conversion of glutamate to α-ketoglutarate using a glutamate-alanine transaminase, and 2 conversion of valine to α-ketoisovalerate via Transaminase C. Not much is known about the regulation of alanine synthesis. The only definite method is the bacterium's ability to repress Transaminase C activity by either valine or leucine see ilvEDA operon. Other than that, alanine biosynthesis does not seem to be regulated. Valine is produced by a four-enzyme pathway. It begins with the condensation of two equivalents of pyruvate catalyzed by acetohydroxy acid synthase yielding α-acetolactate. This is catalyzed by acetohydroxy isomeroreductase. The third step is the dehydration of α, β-dihydroxyisovalerate catalyzed by dihydroxy acid dehydrase. In the fourth and final step, the resulting α-ketoisovalerate undergoes transamination catalyzed either by an alanine-valine transaminase or a glutamate-valine transaminase. Valine biosynthesis is subject to feedback inhibition in the production of acetohydroxy acid synthase. The leucine synthesis pathway diverges from the valine pathway beginning with α-ketoisovalerate. α-Isopropylmalate synthase catalyzes this condensation with acetyl CoA to produce α-isopropylmalate. An isomerase converts α-isopropylmalate to β-isopropylmalate. The final step is the transamination of the α-ketoisocaproate by the action of a glutamate-leucine transaminase. Leucine, like valine, regulates the first step of its pathway by inhibiting the action of the α-Isopropylmalate synthase. The genes that encode both the dihydroxy acid dehydrase used in the creation of α-ketoisovalerate and Transaminase E, as well as other enzymes are encoded on the ilvEDA operon. This operon is bound and inactivated by valine , leucine , and isoleucine. Isoleucine is not a direct derivative of pyruvate, but is produced by the use of many of the same enzymes used to produce valine and, indirectly, leucine. When one of these amino acids is limited, the gene furthest from the amino-acid binding site of this operon can be transcribed. When a second of these amino acids is limited, the next-closest gene to the binding site can be transcribed, and so forth. The commercial production of amino acids usually relies on mutant bacteria that overproduce individual amino acids using glucose as a carbon source. Some amino acids are produced by enzymatic conversions of synthetic intermediates. Aspartic acid is produced by the addition of ammonia to fumarate using a lyase. See Template:Leucine metabolism in humans — this diagram does not include the pathway for β-leucine synthesis via leucine 2,3-aminomutase. Contents move to sidebar hide. Article Talk. Read Edit View history. Tools Tools. What links here Related changes Upload file Special pages Permanent link Page information Cite this page Get shortened URL Download QR code Wikidata item. Download as PDF Printable version. The set of biochemical processes by which amino acids are produced. For the non-biological synthesis of amino acids, see Strecker amino acid synthesis. Demand Media. Retrieved 28 July Annual Review of Microbiology. doi : PMID The physiology and biochemistry of prokaryotes 3rd ed. New York: Oxford Univ. ISBN Journal of Molecular Biology. PMC Principles of Biochemistry 3rd ed. New York: W. Lehninger, Principles of Biochemistry 3rd ed. New York: Worth Publishing. Microbial Biotechnology. ISSN The Journal of Biological Chemistry. Amino Acids. S2CID The Biosynthesis of Histidine and Its Regulation. Archived from the original on 9 December Retrieved 29 April International Journal of Biochemistry. In Wendisch VF ed. Amino acid biosynthesis: pathways, regulation, and metabolic engineering. Berlin: Springer. Annual Review of Biochemistry. Ullmann's Encyclopedia of Industrial Chemistry. Weinheim: Wiley-VCH. Gene expression. Bacterial Archaeal Eukaryotic. Transcription factor RNA polymerase Promoter. Ribosome Transfer RNA tRNA Ribosome-nascent chain complex RNC Post-translational modification. Epigenetic imprinting Transcriptional Gene regulatory network cis-regulatory element lac operon Post-transcriptional sequestration P-bodies alternative splicing microRNA Translational Post-translational reversible irreversible. François Jacob Jacques Monod. Metabolism , catabolism , anabolism. Metabolic pathway Metabolic network Primary nutritional groups. Purine metabolism Nucleotide salvage Pyrimidine metabolism Purine nucleotide cycle. Pentose phosphate pathway Fructolysis Polyol pathway Galactolysis Leloir pathway. Glycosylation N-linked O-linked. |
Video
137-Synthesis of Essential Amino Acids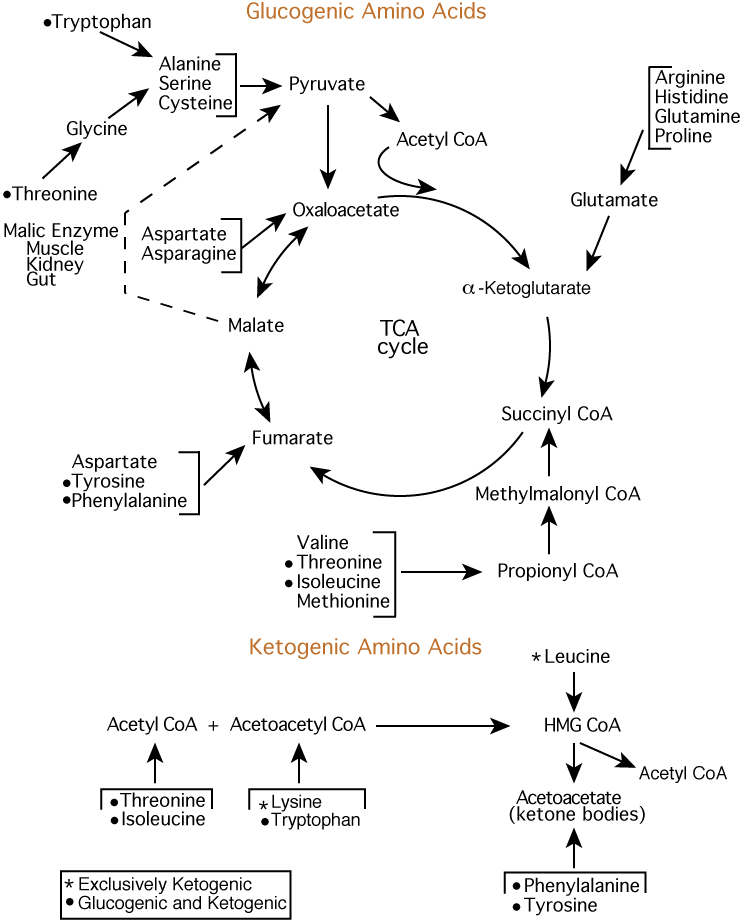
Es ist die lustige Phrase