Increase thermogenic potential -
Because currently available anti-obesity medications are often limited by their psychological or cardiovascular side effects, specific targeting of adipose tissue is needed to remove any potential side effects. Most small molecules, including berberine, butein and β3-AR agonists, have shown effects on the neuronal system, thus suggesting that they might have unwanted actions on the cardiovascular system or neuronal tissues.
By increasing the concentration of the drugs in specific tissues, but not in others, targeted drug delivery can avoid the interaction of thermogenic small molecules with healthy tissues, thereby overcoming the downfalls of conventional methods of drug delivery.
Indeed, a recent discovery by the Langer group has shown that nanoparticle drug delivery methods targeting adipose tissues can be effective for obesity and insulin resistance without drug accumulation in other tissues. In addition, targeting CNS or mimicking outflow of PNS to activate brown fat or WAT browning can have therapeutic potential.
Autonomic hypothalamic innervation and peripheral temperature-sensitive neurons are involved in BAT activation and energy expenditure.
Cancer cachexia is an atrophy of muscle and adipose tissue in cancer patients. This condition can be easily observed in cancer patients and is one of the main causes of decreased survival rates and survival periods in cancer patients. Neutralization of PTHrP in cancer-bearing mice has abrogated the WAT browning effect by cancer.
Similar to PTHrP, anti-inflammatory treatments can also reduce the thermogenic activity of adipose tissues. As such, current approaches for diminishing symptoms of cancer cachexia rely on anti-inflammatory treatments. In the future, more specific targets, such as PTHrP and IL-6, should be investigated to alleviate cancer cachexia.
Because numerous genetic factors and small molecules have been reported to have effects on WAT browning, approaches that reduce thermogenic adipocytes should be considered for cancer patients. How can we better and effectively activate BAT?
With only a handful of thermogenic small molecules being available, a few immediate strategies can be used to achieve better treatment effects. First, chemical modifications can be made to increase solubility, enhance targeted delivery, and improve controlled release.
For instance, structure—activity relationship studies by chemical optimization can bring about thermogenic small molecules that are more effective against obesity and metabolic diseases.
For example, optimized sirtuin inhibitors based on resveratrol have been developed, and their efficacies have been tested in diabetic animals.
Modifications, such as PEGylation, encapsulation with nanoparticles, and various other approaches executed for cancer, could also be applied for diabetes treatment.
Targeted delivery to regions, such as hypothalamic sites, brown adipose tissues, and white adipose tissues, can further reduce dosages and side effects in healthy tissues. This approach can also reduce the fluctuation of chemical levels in the circulation.
The field of drug delivery has advanced markedly in the past few decades. Collaboration is needed to increase the pharmacokinetics of thermogenic small molecules in the near future.
Second, combinatorial compounds are currently being used for various diseases. Combinatory treatments with two or more drugs with different mechanisms may thus increase the beneficial metabolic effects. For example, GLP-1 agonist, liraglutide, and melanocortin receptor agonist, RM, have been shown to have additive metabolic benefits in diet-induced obese mice.
The amounts of BAT, BMI, environments local temperatures, exercise, and food and genomes can vary widely among individuals.
Therefore, unique approaches can be made for each patient. To achieve this, improved diagnosis of BAT activation in humans preferably with non-invasive approaches and identification of biomarkers would be required to dissect the differences needed for personalized medicine.
Finally, aside from focusing on the chemistry of small molecules, small molecules can also be used as tools to identify new molecular targets for therapeutic intervention and thus provide novel insights on the plasticity of adipocytes.
Using the same concept, PPARγ, MyoD and Sirt1 have been identified as molecular targets of a thiazolidinedione, suberanilohydroxamic acid and resveratrol, respectively. These molecules have been highlighted as targets for the therapeutic intervention of metabolic diseases and have offered novel insights into the biology of such diseases.
Likewise, the identification of Prdm4 by using butein also emphasizes the utility of small molecules in the better understanding BAT physiology. Therefore, identification of better small molecules could provide new insights into thermogenic adipocytes.
These molecules can thus be used as alternative therapeutic targets to develop interventions against obesity and metabolic dysregulation.
Recent studies of metabolism have focused on understanding the biology of BAT. This adipose tissue utilizes glucose and fatty acids as energy sources to burn calories and generate heat in response to cold exposure. However, further research is needed to reveal the significance of BAT and WAT browning in humans and its potential applications in human metabolic diseases.
Hill JO, Peters JC. Environmental contributions to the obesity epidemic. Science ; : — Article CAS PubMed Google Scholar. Buysschaert B, Aydin S, Morelle J, Hermans MP, Jadoul M, Demoulin N.
Weight loss at a high cost: orlistat-induced late-onset severe kidney disease. Diabetes Metab ; 42 : 62— Filippatos TD, Derdemezis CS, Gazi IF, Nakou ES, Mikhailidis DP. Orlistat-associated adverse effects and drug interactions—a critical review. Drug Safety ; 31 : 53— Kose M, Emet S, Akpinar TS, Ilhan M, Gok AFK, Dadashov M et al.
An unexpected result of obesity treatment: orlistat-related acute pancreatitis. Case Rep Gastroenterol ; 9 : — Article PubMed PubMed Central Google Scholar. Douglas A, Douglas JG, Robertson CE, Munro JF. Plasma phentermine levels, weight loss and side-effects.
Int J Obes ; 7 : — CAS PubMed Google Scholar. Rosen ED, Spiegelman BM. What we talk about when we talk about fat. Cell ; : 20— Article CAS PubMed PubMed Central Google Scholar.
Harms M, Seale P. Brown and beige fat: development, function and therapeutic potential. Nat Med ; 19 : — Cannon B, Nedergaard J. Brown adipose tissue: function and physiological significance. Physiol Rev ; 84 : — van Marken Lichtenbelt WD, Vanhommerig JW, Smulders NM, Drossaerts JM, Kemerink GJ, Bouvy ND et al.
Cold-activated brown adipose tissue in healthy men. N Engl J Med ; : — Cypess AM, Weiner LS, Roberts-Toler C, Franquet Elía E, Kessler SH, Kahn PA et al. Activation of human brown adipose tissue by a beta 3-adrenergic receptor agonist. Cell Metab ; 21 : 33— Symonds ME, Pope M, Budge H.
The ontogeny of brown adipose tissue. Annu Rev Nutr ; 35 : — Zhang Z, Zhang H, Li B, Meng X, Wang J, Zhang Y et al. Berberine activates thermogenesis in white and brown adipose tissue. Nat Commun ; 5 : Song NJ, Choi S, Rajbhandari P, Chang SH, Kim S, Vergnes L et al.
Prdm4 induction by the small molecule butein promotes white adipose tissue browning. Nat Chem Biol ; 12 : — van Dam AD, Nahon KJ, Kooijman S, van den Berg SM, Kanhai AA, Kikuchi T et al. Salsalate activates brown adipose tissue in mice. Diabetes ; 64 : — Maeda H, Hosokawa M, Sashima T, Funayama K, Miyashita K.
Fucoxanthin from edible seaweed, Undaria pinnatifida, shows antiobesity effect through UCP1 expression in white adipose tissues.
Biochem Bioph Res Co ; : — Article CAS Google Scholar. Ohno H, Shinoda K, Spiegelman BM, Kajimura S. PPAR gamma agonists induce a white-to-brown fat conversion through stabilization of PRDM16 protein. Cell Metab ; 15 : — Nicholls DG. The physiological regulation of uncoupling proteins.
Biochim Biophys Acta ; : — Ricquier D. Uncoupling protein 1 of brown adipocytes, the only uncoupler: a historical perspective. Front Endocrinol ; 2 : Saito M, Okamatsu-Ogura Y, Matsushita M, Watanabe K, Yoneshiro T, Nio-Kobayashi J et al.
High incidence of metabolically active brown adipose tissue in healthy adult humans effects of cold exposure and adiposity. Diabetes ; 58 : — Huang SG. Binding of fatty acids to the uncoupling protein from brown adipose tissue mitochondria. Arch Biochem Biophys ; : — Beck V, Jabůrek M, Demina T, Rupprecht A, Porter RK, Jezek P et al.
Polyunsaturated fatty acids activate human uncoupling proteins 1 and 2 in planar lipid bilayers. FASEB J ; 21 : — Minokoshi Y, Kim YB, Peroni OD, Fryer LG, Müller C, Carling D et al.
Leptin stimulates fatty-acid oxidation by activating AMP-activated protein kinase. Nature ; : — Chouchani ET, Kazak L, Jedrychowski MP, Lu GZ, Erickson BK, Szpyt J et al. Mitochondrial ROS regulate thermogenic energy expenditure and sulfenylation of UCP1. Sears IB, MacGinnitie MA, Kovacs LG, Graves RA.
Differentiation-dependent expression of the brown adipocyte uncoupling protein gene: regulation by peroxisome proliferator-activated receptor gamma. Mol Cell Biol ; 16 : — Seale P, Kajimura S, Yang W, Chin S, Rohas LM, Uldry M et al. Transcriptional control of brown fat determination by PRDM Cell Metab ; 6 : 38— Kajimura S, Seale P, Kubota K, Lunsford E, Frangioni JV, Gygi SP et al.
Puigserver P, Wu Z, Park CW, Graves R, Wright M, Spiegelman BM. A cold-inducible coactivator of nuclear receptors linked to adaptive thermogenesis. Cell ; 92 : — Lin J, Wu PH, Tarr PT, Lindenberg KS, St-Pierre J, Zhang CY et al. Defects in adaptive energy metabolism with CNS-linked hyperactivity in PGC-1alpha null mice.
Cell ; : — Uldry M, Yang W, St-Pierre J, Lin J, Seale P, Spiegelman BM. Complementary action of the PGC-1 coactivators in mitochondrial biogenesis and brown fat differentiation. Cell Metab ; 3 : — Kazak L, Chouchani ET, Jedrychowski MP, Erickson BK, Shinoda K, Cohen P et al.
A creatine-driven substrate cycle enhances energy expenditure and thermogenesis in beige fat. Ukropec J, Anunciado RP, Ravussin Y, Hulver MW, Kozak LP. J Biol Chem ; : — Long JZ, Svensson KJ, Bateman LA, Lin H, Kamenecka T, Lokurkar IA et al.
The secreted enzyme PM20D1 regulates lipidated amino acid uncouplers of mitochondria. Kajimura S, Seale P, Tomaru T, Erdjument-Bromage H, Cooper MP, Ruas JL et al.
Gene Dev ; 22 : — Seale P, Conroe HM, Estall J, Kajimura S, Frontini A, Ishibashi J et al. Prdm16 determines the thermogenic program of subcutaneous white adipose tissue in mice. J Clin Invest ; : 96— Cohen P, Levy JD, Zhang Y, Frontini A, Kolodin DP, Svensson KJ et al. Ablation of PRDM16 and beige adipose causes metabolic dysfunction and a subcutaneous to visceral fat switch.
Seale P, Bjork B, Yang W, Kajimura S, Chin S, Kuang S et al. Ohno H, Shinoda K, Ohyama K, Sharp LZ, Kajimura S. EHMT1 controls brown adipose cell fate and thermogenesis through the PRDM16 complex. Villanueva CJ, Vergnes L, Wang J, Drew BG, Hong C, Tu Y et al.
Adipose subtype-selective recruitment of TLE3 or Prdm16 by PPAR gamma specifies lipid storage versus thermogenic gene programs. Cell Metab ; 17 : — Iida S, Chen W, Nakadai T, Ohkuma Y, Roeder RG. PRDM16 enhances nuclear receptor-dependent transcription of the brown fat- specific Ucp1 gene through interactions with mediator subunit MED1.
Gene Dev ; 29 : — Harms MJ, Lim HW, Ho Y, Shapira SN, Ishibashi J. PRDM16 binds MED1 and controls chromatin architecture to determine a brown fat transcriptional program.
Genes Dev ; 29 : — Kong X, Banks A, Liu T, Kazak L, Rao RR, Cohen P et al. IRF4 is a key thermogenic transcriptional partner of PGC-1 alpha. Cell ; : 69— Davis KE, Moldes M, Farmer SR. The forkhead transcription factor FoxC2 inhibits white adipocyte differentiation.
Gerin I, Bommer GT, Lidell ME, Cederberg A, Enerback S, Macdougald OA. On the role of FOX transcription factors in adipocyte differentiation and insulin-stimulated glucose uptake.
You W, Fan L, Duan D, Tian L, Dang X, Wang C et al. Foxc2 over-expression in bone marrow mesenchymal stem cells stimulates osteogenic differentiation and inhibits adipogenic differentiation.
Mol Cell Biochem ; : — Cederberg A, Grønning LM, Ahrén B, Taskén K, Carlsson P, Enerbäck S. FOXC2 is a winged helix gene that counteracts obesity, hypertriglyceridemia, and diet-induced insulin resistance.
Dempersmier J, Sambeat A, Gulyaeva O, Paul SM, Hudak CS, Raposo HF et al. Cold-inducible Zfp activates UCP1 transcription to promote browning of white fat and development of brown fat. Mol Cell ; 57 : — Sambeat A, Gulyaeva O, Dempersmier J, Tharp KM, Stahl A, Paul SM et al.
LSD1 interacts with Zfp to promote UCP1 transcription and brown fat program. Cell Rep ; 15 : — Boström P, Wu J, Jedrychowski MP, Korde A, Ye L, Lo JC et al. A PGC1-alpha-dependent myokine that drives brown-fat-like development of white fat and thermogenesis.
Lee P, Linderman JD, Smith S, Brychta RJ, Wang J, Idelson C et al. Irisin and FGF21 are cold-induced endocrine activators of brown fat function in humans. Cell Metab ; 19 : — Jedrychowski MP, Wrann CD, Paulo JA, Gerber KK, Szpyt J, Robinson MM et al. Detection and quantitation of circulating human irisin by tandem mass spectrometry.
Cell Metab ; 22 : — Fisher FM, Maratos-Flier E. Understanding the physiology of FGF Annu Rev Physiol ; 78 : — Fisher FM, Kleiner S, Douris N, Fox EC, Mepani RJ, Verdeguer F et al. FGF21 regulates PGC-1 alpha and browning of white adipose tissues in adaptive thermogenesis.
Gene Dev ; 26 : — Nguyen KD, Qiu Y, Cui X, Goh YP, Mwangi J, David T et al. Alternatively activated macrophages produce catecholamines to sustain adaptive thermogenesis.
Lee MW, Odegaard JI, Mukundan L, Qiu Y, Molofsky AB, Nussbaum JC et al. Activated type 2 innate lymphoid cells regulate beige fat biogenesis. Cell ; : 74— Abd El-Wahab AE, Ghareeb DA, Sarhan EE, Abu-Serie MM, El Demellawy MA.
In vitro biological assessment of Berberis vulgaris and its active constituent, berberine: antioxidants, anti-acetylcholinesterase, anti-diabetic and anticancer effects.
BMC Complement Altern Med ; 13 : Cernáková M, Kost'álová D, Kettmann V, Plodová M, Tóth J, Drímal J. Potential antimutagenic activity of berberine, a constituent of Mahonia aquifolium.
BMC Complement Altern Med ; 2 : 2. Brown PN, Roman MC. Determination of hydrastine and berberine in goldenseal raw materials, extracts, and dietary supplements by high-performance liquid chromatography with UV: collaborative study.
J AOAC Int ; 91 : — Hashida K, Tabata M, Kuroda K, Otsuka Y, Kubo S, Makino S et al. Phenolic extractives in the trunk of Toxicodendron vernicifluum : chemical characteristics, contents and radial distribution.
J Wood Sci ; 60 : — Song NJ, Yoon HJ, Kim KH, Jung SR, Jang WS, Seo CR et al. Butein is a novel anti-adipogenic compound. J Lipid Res ; 54 : — Sung J, Lee J. Anti-inflammatory activity of butein and luteolin through suppression of NF kappa B activation and induction of heme oxygenase J Med Food ; 18 : — Zhang L, Chen W, Li X.
FEBS Lett ; : — Usman MG, Rafii MY, Ismail MR, Malek MA, Latif MA. Capsaicin and dihydrocapsaicin determination in chili pepper genotypes using ultra-fast liquid chromatography.
Molecules ; 19 : — Zhang LL, Yan Liu D, Ma LQ, Luo ZD, Cao TB, Zhong J et al. Activation of transient receptor potential vanilloid type-1 channel prevents adipogenesis and obesity. Circ Res ; : — Luo Z, Ma L, Zhao Z, He H, Yang D, Feng X et al. TRPV1 activation improves exercise endurance and energy metabolism through PGC-1 alpha upregulation in mice.
Cell Res ; 22 : — Joo JI, Kim DH, Choi JW, Yun JW. Proteomic analysis for antiobesity potential of capsaicin on white adipose tissue in rats fed with a high fat diet. J Proteome Res ; 9 : — Ludy MJ, Moore GE, Mattes RD. The effects of capsaicin and capsiate on energy balance: critical review and meta-analyses of studies in humans.
Chem Senses ; 37 : — Andero R, Ressler KJ. Fear extinction and BDNF: translating animal models of PTSD to the clinic. Genes Brain Behav ; 11 : — Liu CY, Chan CB, Ye KQ. Transl Neurodegener ; 5 : 2. Zeng Y, Wang X, Wang Q, Liu S, Hu X, McClintock SM.
Small molecules activating TrkB receptor for treating a variety of CNS disorders. CNS Neurol Disord Drug Targets ; 12 : — Chan CB, Tse MC, Liu X, Zhang S, Schmidt R, Otten R et al.
Activation of muscular TrkB by its small molecular agonist 7,8-dihydroxyflavone sex-dependently regulates energy metabolism in diet-induced obese mice.
Chem Biol ; 22 : — Peng J, Yuan JP, Wu CF, Wang JH. Fucoxanthin, a marine carotenoid present in brown seaweeds and diatoms: metabolism and bioactivities relevant to human health. Mar Drugs ; 9 : — Maeda H, Hosokawa M, Sashima T, Murakami-Funayama K, Miyashita K. Anti-obesity and anti-diabetic effects of fucoxanthin on diet-induced obesity conditions in a murine model.
Mol Med Rep ; 2 : — Kumar SR, Hosokawa M, Miyashita K. Fucoxanthin: a marine carotenoid exerting anti-cancer effects by affecting multiple mechanisms. Mar Drugs ; 11 : — Maeda H, Tsukui T, Sashima T, Hosokawa M, Miyashita K. Seaweed carotenoid, fucoxanthin, as a multi-functional nutrient.
Asia Pac J Clin Nutr ; 17 : — Rosen ED, MacDougald OA. Adipocyte differentiation from the inside out. Nat Rev Mol Cell Biol ; 7 : — Fukui Y, Masui S, Osada S, Umesono K, Motojima K. A new thiazolidinedione, NC, which is a weak PPAR-gamma activator, exhibits potent antidiabetic effects and induces uncoupling protein 1 in white adipose tissue of KKAy obese mice.
Diabetes ; 49 : — Petrovic N, Walden TB, Shabalina IG, Timmons JA, Cannon B, Nedergaard J. Chronic peroxisome proliferator-activated receptor gamma PPAR gamma activation of epididymally derived white adipocyte cultures reveals a population of thermogenically competent, UCP1-containing adipocytes molecularly distinct from classic brown adipocytes.
Wilson-Fritch L, Nicoloro S, Chouinard M, Lazar MA, Chui PC, Leszyk J et al. Mitochondrial remodeling in adipose tissue associated with obesity and treatment with rosiglitazone.
J Clin Invest ; : — Moisan A, Lee YK, Zhang JD, Hudak CS, Meyer CA, Prummer M et al. White-to-brown metabolic conversion of human adipocytes by JAK inhibition.
Nat Cell Biol ; 17 : 57— Andersson ER, Lendahl U. Therapeutic modulation of Notch signalling—are we there yet? Nat Rev Drug Discov ; 13 : — Bi PP, Kuang SH. Notch signaling as a novel regulator of metabolism.
Trends Endocrin Met ; 26 : — Bi P, Shan T, Liu W, Yue F, Yang X, Liang XR et al. Inhibition of Notch signaling promotes browning of white adipose tissue and ameliorates obesity.
Nat Med ; 20 : — Anderson K, Wherle L, Park M, Nelson K, Nguyen L. Salsalate, an old, inexpensive drug with potential new indications: a review of the evidence from 3 recent studies.
Am Health Drug Benefits ; 7 : — PubMed PubMed Central Google Scholar. Rumore MM, Kim KS. Potential role of salicylates in type 2 diabetes.
Ann Pharmacother ; 44 : — Goldfine AB, Fonseca V, Jablonski KA, Pyle L, Staten MA, Shoelson SE. The effects of salsalate on glycemic control in patients with type 2 diabetes: a randomized trial.
Ann Intern Med ; : — Meex RCR, Phielix E, Moonen-Kornips E, Schrauwen P, Hesselink MKC. Stimulation of human whole-body energy expenditure by salsalate is fueled by higher lipid oxidation under fasting conditions and by higher oxidative glucose disposal under insulin-stimulated conditions.
J Clin Endocr Metab ; 96 : — Cao W, Medvedev AV, Daniel KW, Collins S. beta-Adrenergic activation of p38 MAP kinase in adipocytes: cAMP induction of the uncoupling protein 1 UCP1 gene requires p38 MAP kinase. Lowell BB, Spiegelman BM. Towards a molecular understanding of adaptive thermogenesis. Jimenez M, Barbatelli G, Allevi R, Cinti S, Seydoux J, Giacobino JP et al.
Eur J Biochem ; : — Bachman ES, Dhillon H, Zhang CY, Cinti S, Bianco AC, Kobika BK et al. betaAR signaling required for diet-induced thermogenesis and obesity resistance.
Wu J, Boström P, Sparks LM, Ye L, Choi JH, Giang AH et al. Beige adipocytes are a distinct type of thermogenic fat cell in mouse and human.
Cinti S, Cancello R, Zingaretti MC, Ceresi E, De Matteis R, Giordano A et al. CL, and cold stress induce heterogeneous expression of UCP1 mRNA and protein in rodent brown adipocytes. J Histochem Cytochem ; 50 : 21— Wang J, Liu R, Wang F, Hong J, Li X, Chen M et al.
Ablation of LGR4 promotes energy expenditure by driving white-to-brown fat switch. Nat Cell Biol ; 15 : — Haas B, Mayer P, Jennissen K, Scholz D, Berriel Diaz M, Bloch W et al. Protein kinase G controls brown fat cell differentiation and mitochondrial biogenesis.
Sci Signal ; 2 : ra Mitschke MM, Hoffmann LS, Gnad T, Scholz D, Kruithoff K, Mayer P et al. Increased cGMP promotes healthy expansion and browning of white adipose tissue. FASEB J ; 27 : — Hoffmann LS, Etzrodt J, Willkomm L, Sanyal A, Scheja L, Fisher AW et al. Stimulation of soluble guanylyl cyclase protects against obesity by recruiting brown adipose tissue.
Nat Commun ; 6 : Kessler RJ, Tyson CA, Green DE. Mechanism of uncoupling in mitochondria: uncouplers as ionophores for cycling cations and protons.
Proc Natl Acad Sci USA ; 73 : — Grundlingh J, Dargan PI, El-Zanfaly M, Wood DM. J Med Toxicol ; 7 : — Petróczi A, Ocampo JA, Shah I, Jenkinson C, New R, James RA et al.
Russian roulette with unlicensed fat-burner drug 2,4-dinitrophenol DNP : evidence from a multidisciplinary study of the internet, bodybuilding supplements and DNP users.
Subst Abuse Treat Prev Policy ; 10 : Tecott LH. Serotonin and the orchestration of energy balance. Cell Metab ; 6 : — Crane JD, Palanivel R, Mottillo EP, Bujak AL, Wang H, Ford RJ et al. Inhibiting peripheral serotonin synthesis reduces obesity and metabolic dysfunction by promoting brown adipose tissue thermogenesis.
Nat Med ; 21 : — Oh CM, Namkung J, Go Y, Shong KE, Kim K, Kim H et al. Regulation of systemic energy homeostasis by serotonin in adipose tissues. Rogatzki MJ, Ferguson BS, Goodwin ML, Gladden LB.
Lactate is always the end product of glycolysis. Front Neurosci ; 9 : Carrière A, Jeanson Y, Berger-Müller S, André M, Chenouard V, Arnaud E et al. Browning of white adipose cells by intermediate metabolites: an adaptive mechanism to alleviate redox pressure.
Diabetes ; 63 : — Roberts LD, Boström P, O'Sullivan JF, Schinzel RT, Lewis GD, Dejam A et al. Beta-aminoisobutyric acid induces browning of white fat and hepatic beta-oxidation and is inversely correlated with cardiometabolic risk factors.
Cell Metab ; 19 : 96— Lundberg JO, Weitzberg E, Gladwin MT. The nitrate-nitrite-nitric oxide pathway in physiology and therapeutics. Nat Rev Drug Discov ; 7 : — Roberts LD, Ashmore T, Kotwica AO, Murfitt SA, Fernandez BO, Fellisch M et al.
Inorganic nitrate promotes the browning of white adipose tissue through the nitrate-nitrite-nitric oxide pathway. Burnstock G. Physiology and pathophysiology of purinergic neurotransmission. Physiol Rev ; 87 : — Schimmel RJ, McCarthy L. Role of adenosine as an endogenous regulator of respiration in hamster brown adipocytes.
Am J Physiol ; : C—C Szillat D, Bukowiecki LJ. Control of brown adipose tissue lipolysis and respiration by adenosine. Am J Physiol ; : E—E Gnad T, Scheibler S, von Kügelgen I, Scheele C, Kilić A, Glöde A et al. Adenosine activates brown adipose tissue and recruits beige adipocytes via A2A receptors.
Guo T, Marmol P, Moliner A, Björnholm M, Zhang C, Shokat KM et al. Adipocyte ALK7 links nutrient overload to catecholamine resistance in obesity. Elife ; 3 : e Ouellet V, Labbé SM, Blondin DP, Phoenix S, Guérin B, Haman F et al.
Brown adipose tissue oxidative metabolism contributes to energy expenditure during acute cold exposure in humans. Zingaretti MC, Crosta F, Vitali A, Guerrieri M, Frontini A, Cannon B et al.
The presence of UCP1 demonstrates that metabolically active adipose tissue in the neck of adult humans truly represents brown adipose tissue. FASEB J ; 23 : — Xue Y, Xu X, Zhang XQ, Farokhzad OC, Langer R. Preventing diet-induced obesity in mice by adipose tissue transformation and angiogenesis using targeted nanoparticles.
Proc Natl Acad Sci USA ; : — Chau YY, Hastie N. Wt1, the mesothelium and the origins and heterogeneity of visceral fat progenitors. Adipocyte ; 4 : — Bartness TJ, Vaughan CH, Song CK. Sympathetic and sensory innervation of brown adipose tissue. Int J Obesity ; 34 : S36—S Protein tends to stimulate diet-induced thermogenesis to a greater extent than either carbohydrates or fat.
For this reason, a higher-protein diet is often recommended for those looking to lose body fat and change their body composition - higher-protein diets not only increase the number of calories you're expending just due to digesting the macronutrient, but high protein intake is also linked to better satiety, meaning those eating higher-protein diets tend to consume fewer calories overall.
Brown adipose tissue is a kind of body fat activated in response to cold exposure. Its primary role is producing heat to help the body maintain the proper temperature, which requires calories. Research has found that brown adipose tissue may also play a role in diet-induced thermogenesis.
Production of gut-related hormones and compounds such as bile acids not only contributes to diet-induced thermogenesis but also activates brown adipose tissue directly, helping to increase thermogenesis overall. Additionally, the thyroid gland plays a role in this connection, with adequate thyroid function needed for activating brown adipose tissue and optimal thermogenesis.
Since morning cold plunges have become popular additions to a lifestyle that supports overall metabolic health, it could be that the activation of brown adipose tissue plays a role in the purported body composition benefits of cold plunging.
Pair that with the fact that diet-induced thermogenesis has been found in studies to be higher in the morning than the evening, and it may lend credibility to why those who eat earlier in the day and participate in biohacking modalities like a morning cold plunge see favorable body composition changes - it may be due to an amplified diet-induced thermogenic response.
The following functional medicine labs can be utilized to assess areas that contribute to diet-induced thermogenesis:. Since adequate digestion and absorption are central to diet-induced thermogenesis, it can be helpful to evaluate the health and function of the GI digestive system through a comprehensive digestive stool analysis.
A healthy gut produces all of the necessary enzymes and compounds needed to digest food, activate brown adipose tissue, and increase metabolic rate in response to the diet; without adequate production of, for example, bile acids, the diet-induced thermogenic response described above may not be as optimal.
Since thyroid function helps modulate thermogenesis as a whole, in addition to the activation of brown adipose tissue, it can be helpful to assess if one's thyroid is working optimally. There's also a close link between thyroid function and bile acids, meaning a full thyroid panel can help provide insight into the bigger picture of how well the metabolic pathways underlying diet-induced thermogenesis are functioning.
Understanding your body fat percentage and the amount of muscle mass you have can be helpful when considering your total daily energy expenditure, calculating the amount of protein and other macronutrients in your diet, and ensuring any weight loss efforts are producing loss of body fat rather than valuable muscle mass.
The ideal nutritional approach to improve thermogenesis would be a diet that meets protein needs or protein-forward nutrition. While many "diets" fit the bill, the diet likely to lead to the highest rate of diet-induced thermogenesis would be a higher protein diet.
Adding in foods such as capsaicin cayenne pepper and green tea may also be helpful, and choosing primarily whole food sources of fats and carbohydrates versus processed foods would be ideal. Many supplements are labeled "thermogenic aids" or "fat burners," with a few ingredients in common that may improve thermogenesis.
However, it's critical to note that many of these supplements marketed as " fat burners " contain amounts far beyond a "single" serving of a given compound and are not well-regulated regarding safety and efficacy.
Below are several supplements supported by literature regarding their impact on thermogenesis. Green tea extract and its catechin content have been linked to increases in energy expenditure and thermogenesis, as well as increased fat oxidation. While some studies suggest that any thermogenic aid has a risk of increased heart rate and blood pressure, green tea extract is one of the safest aids, with a daily intake of mg or less in a supplemental form deemed safe.
Caffeine intake has been linked to increased thermogenesis and brown adipose tissue activation. However, many "thermogenic aid" supplements have high amounts of caffeine, up to four times the amount you'd get from drinking one cup of coffee.
Higher caffeine intake also increases the risk of short-term elevated heart rates, palpitations, blood pressure, and anxiety. For those with higher cardiovascular risk, caffeine may not be the best thermogenic aid to consider.
Capsaicin supplements have been linked to weight loss through the effect of capsaicin on thermogenesis. However, the impact may be seen more in lean individuals, as studies have shown inconsistent results in obese populations. Up to 6mg taken daily for 12 weeks has been shown to have favorable effects on body composition, especially when paired with a healthy diet and exercise plan.
In addition to diet composition, studies also show that eating slowly and ensuring that you're chewing your food thoroughly helps to increase the rate of diet-induced thermogenesis. So if you're working on a body composition goal, make sure you're taking the time to pause, slow down, and intentionally chew your meal.
This helps with the digestive process and production of enzymes and hormones important for digestion and translates to expending more calories while digesting your food.
Diet-induced thermogenesis refers to the impact your food and digestion can have on the calories expended each day. The amount of brown adipose tissue BAT one has can correlate with more efficient diet-induced thermogenesis, and diets higher in protein tend to impart a higher percentage of diet-induced thermogenesis to total daily energy expenditure, making them ideal for those looking to lose body fat percentage.
Last, diet-induced thermogenesis is more efficient earlier in the day than at night, which is an important consideration for meal timing and planning as part of a larger holistic approach to one's nutrition.
Documents Tab. Redesigned Patient Portal. Simplify blood panel ordering with Rupa's Panel Builder. Sign in. Sign in Sign up free. Subscribe for free to keep reading! If you are already subscribed, enter your email address to log back in.
Are you a healthcare practitioner? Yes No. Search All Content Magazine Podcasts Lab Companies Lab Tests Live Classes Bootcamps Health Categories. Basic Lab Markers. Case Studies. GI Health.
Brown Increase thermogenic potential is a healthy type of fat that is actually darker in color. Once thought pogential only thermogebic in babies, researchers now believe adults Increase thermogenic potential it too. Brown fat has energy that might be harnessed for better health. You may be surprised to learn that the fat in your body is made up of different colors. Scientists have identified both white and brown fat. The brown color is also sometimes referred to as inducible brown adipose tissue BAT.Increase thermogenic potential -
This means that taking a mg caffeine pill would burn an additional 15 calories over the course of a day 4.
Human and animal studies show that doses of 1. Green tea contains two compounds that have thermogenic effects: caffeine and epigallocatechin gallate EGCG 5 , 6. As noted above, caffeine stimulates the release of adrenaline, which boosts metabolism and increases fat burning. EGCG enhances these effects by slowing the breakdown of adrenaline so that its impact is amplified 6 , 7.
One review found that overweight or obese people who consumed green tea supplements daily for at least 12 weeks lost only 0. However, a different review found that individuals who took green tea supplements for the same time period experienced an average weight loss of 2.
More research is needed to better understand how green tea affects metabolism and body composition. Capsaicin is the molecule that makes chili peppers spicy — the spicier the pepper, the more capsaicin it contains.
Like caffeine, capsaicin stimulates the release of adrenaline, which speeds up metabolism and causes your body to burn more calories and fat It also reduces appetite, making you eat fewer calories. Together, these effects make capsaicin a powerful thermogenic substance A review of 20 studies found that capsaicin supplements can boost metabolism by about 50 calories per day, which could lead to significant weight loss over time Another study showed that dieters taking 2.
Supplementing with 6 mg of capsaicin daily has also been linked to reductions in belly fat over a three-month period However, there is some evidence that your body can adapt to capsaicin, reducing these effects over time Garcinia cambogia is a tropical fruit whose extracts are often used in weight loss supplements.
It contains a compound called hydroxycitric acid HCA that can block the activity of the enzyme ATP citrate lyase, which is involved in the formation of body fat This is a difference of roughly 2 pounds 0.
More studies are needed to understand whether garcinia cambogia supplements are effective for weight loss or reducing body fat. Yohimbine is a chemical derived from the bark of the African yohimbe tree, and is commonly taken as a thermogenic supplement.
It works by increasing the activity of several hormones, including adrenaline, noradrenaline and dopamine, which could theoretically boost fat metabolism 22 , The effectiveness of yohimbine for fat loss has not been researched much, but early results are promising.
Yohimbine may be especially effective for weight loss when combined with exercise, since it has been shown to boost fat burning during and after aerobic exercise At present, there is not enough research to determine whether yohimbine truly helps burn body fat.
Bitter orange, a type of citrus fruit , contains synephrine, a compound that is a natural stimulant, similar in structure to ephedrine. While ephedrine has been banned in the United States due to reports of sudden heart-related deaths, synephrine has not been found to have the same effects and is considered safe to use in supplements Taking 50 mg of synephrine has been shown to increase metabolism and burn an additional 65 calories per day, which could potentially help people lose weight over time A review of 20 studies using bitter orange alone or in combination with other herbs found that it significantly increased metabolism and weight loss when taken daily for 6—12 weeks Since many substances have thermogenic effects, companies often combine several of them in one supplement, hoping for greater weight loss effects.
Studies show that these blended supplements provide an extra metabolism boost, especially when combined with exercise. However, there have not been many studies to determine whether they reduce body fat 29 , 30 , 31 , One eight-week study found that overweight and obese dieters who took a daily supplement containing green tea extract, capsaicin and caffeine lost an additional pound 0.
Yet, more research is needed Popular thermogenic supplements include caffeine, green tea, capsaicin, garcinia cambogia , yohimbine and bitter orange. These substances can boost metabolism, increase fat burning and reduce appetite, but the effects are relatively small.
The β 3 -AR has emerged as a leading molecular target for the activation of brown or beige adipocytes 18 , Rodent and human adipose tissue display different β-AR expression profiles.
In mouse, the β 3 -AR is highly expressed in both BAT and WAT with several-fold higher abundance than the β 1 -AR, whereas in the adult human, the β 3 -AR is only expressed in BAT.
In human WAT, β 1 -AR has been reported to be fold more abundant than β 3 -AR The β 3 -AR subtypes also differ in their potency toward various ligands, G-protein coupling, and desensitization. The binding affinity of NE to β 3 -AR was reported to be in the low micromolar range, while the potency for cAMP accumulation is in the low nanomolar range when measured in intact cells, suggesting that the coupling efficacy to adenylyl cyclase of β 3 -AR was higher than that of β 1 -AR 52 , In addition, β 1 -AR desensitizes more rapidly than β 3 -AR upon exposure to agonists.
This led to the hypothesis that β 1 -AR may mediate the NE response to low levels of sympathetic stimulation. On the other hand, the activation of β 3 -AR may require higher levels of sympathetic stimulation, but once activated this receptor is likely to deliver a more sustained effect It is important to note that circulating NE levels are generally in the low nanomolar concentration range, and it is likely that NE concentrations at the synaptic clefts will be much higher during cold response, providing sufficiently high local concentrations in BAT to enable local activation of β 3 -AR in spite of the low plasma concentration.
Recently, several studies have shown that systemic administration of adrenergic activators, such as isoproterenol ISO and ephedrine, fail to elicit BAT activation in man 55 — To properly interpret these clinical data, it is crucial to understand if the plasma concentrations of the adrenergic agonists used reached a sufficiently high concentration to enable activation of lipolysis and thermogenesis in human BAT.
Norepinephrine-mediated β-AR activation results in an increased intracellular cAMP concentration, which in turn stimulates lipolysis in brown adipocytes via activation of the protein kinase A pathway.
DAG is then hydrolyzed by hormone-sensitive lipase HSL to monoacylglycerol and, subsequently glycerol, with a fatty acid released at each stage. Intracellular FFAs are the direct activators of UCP1 In humans, the BAT radiodensity which is indicative of intracellular TG stores is inversely correlated with NST, strongly suggesting that depletion of intracellular TG occurs during cold exposure.
In rats, inhibition of lipolysis by nicotinic acid-mediated GPRa agonism significantly reduced the oxidative capacity of iBAT in response to cold, again suggesting a key role for lipolysis in UCP1-mediated thermogenesis In brown adipocytes, glucose uptake is mostly mediated by glucose transporter 1 GLUT1 and glucose transporter 4 GLUT4 and subsequently stored as glycogen or converted to lactate through anaerobic glycolysis.
As shown in Figure 3 , FFAs are transported into the cell by cluster of differentiation 36 CD36 and TGs are subsequently synthesized through re-esterification by a series of enzymes, including glycerolphosphate acyltransferase GPAT and diacylglycerol O-acyltransferases DGATs The process of recruitment of new brown or beige adipocytes and the maintenance of mature adipocyte function during acute and chronic cold acclimation are subject to complex transcriptional and hormonal regulation, for example, by PPARγ, bone morphogenic proteins BMPs , the thyroid axis, and FGF These regulatory mechanisms have been extensively reviewed elsewhere 8 , 47 , 48 , The functional activity of BAT is reduced by chronic warm acclimation, old age, obesity, and diabetes.
Old age and lack of cold challenge also reduce the thermogenic activity of the brown adipocytes Brown adipocytes isolated from guinea pigs housed at 30°C appear unilocular, although these cells appear to retain their brown adipocyte identity and are able to respond to NE with a robust increase of thermogenesis, which is not the case for adipocytes isolated from WAT In humans, BAT activity is inversely correlated with age, fat mass, and BMI.
Insulin-stimulated glucose uptake is also compromised in the BAT of diabetic individuals Recent reports that both chronic cold acclimation and weight loss can enhance BAT activity in humans are encouraging 27 , 69 , 70 , indicating that reduced BAT function may be restored. In this respect, adipocytes or adipose precursor cells are highly plastic and able to adapt to the functional needs, as has been shown in rodent using lineage tracing experiments The TZDs [represented by rosiglitazone Avandia and pioglitazone Actos ] are a chemical class of PPARγ agonists used as insulin sensitizers for the treatment of T2D The primary site of action of the TZDs is adipose tissue, where they improve several functional aspects, including the uptake and storage of plasma NEFA.
They also increase FFA mobilization under fasting conditions and enhance postprandial suppression of FFA mobilization by insulin Thiazolidinediones have been shown to increase UCP1 expression and BAT mass in rodents.
Rosiglitazone has been shown in vitro by several laboratories to induce UCP1 in rodent brown adipocytes and differentiated adipose stem cells 74 — In addition, chronic treatment of human subcutaneous adipose stem cells with rosiglitazone upregulated several components of the mitochondrial electron transport chain, which is consistent with what has been observed in human The UCP1 protein in human adipocytes differentiated in vitro in the presence of rosiglitazone is only functional when cells have been allowed to differentiate for a longer time than is typically reported in mouse studies An increase in the oxygen consumption rate OCR of human adipocytes differentiated in vitro in the presence of rosiglitazone could only be observed when the cells were stimulated with isoproterenol or in the presence of exogenously provided FFAs.
The increase of OCR in these beige cells is completely UCP1 dependent, as UCP1 knock-down abolishes the effect Various in vivo rodent models of insulin resistance have been used to show that the TZDs increase UCP1 mRNA in iBAT and overall iBAT weight.
However, this is not associated with a subsequent increase in thermogenesis or whole-body EE. In addition, TZD treatment leads to the brown adipocytes becoming lipid filled 79 — This led to the hypothesis that obese and diabetic animals or humans could first be primed by a PPARγ agonist to expand the BAT capacity, followed by its activation via a β-AR agonist.
Synergistic effects on EE and bodyweight reduction were indeed observed in this single study However, a later study using rosiglitazone followed by acute cold exposure failed to reproduce these effects A possible explanation for the lack of increased EE in spite of increased TG storage, UCP1 expression and BAT mass induced upon PPARγ activation by rosiglitazone 84 may be the downregulation of β 3 -AR and iodothyronine deiodinase type II DIO2 expression caused by TZD treatment 85 , In addition, as COOH belongs to a different structural class of PPARγ agonists to the TZDs, it is not unlikely that different outcomes could be observed between the two compounds.
In humans, 12 weeks of pioglitazone treatment has been shown to generate a small increase in UCP1 mRNA levels in subcutaneous adipose tissue However, combined pioglitazone and ephedrine treatment for 12 weeks in obese human subjects failed to deliver significant bodyweight reduction When comparing the observed outcomes of studies employing PPARγ agonists, it must be kept in mind that even structurally closely related compounds may exhibit very different PPARγ-dependent pharmacodynamic profiles.
This is, in part, due to ligand-dependent modulation of the PPARγ protein that leads to recruitment of different coactivator and corepressor proteins, in turn resulting in a unique transcriptional profile for each compound Since the identification of PPARγ as the molecular target of the TZDs 90 , significant effort has been invested by the pharmaceutical industry in PPARγ agonist drug discovery 91 — 96 albeit largely without commercial success Despite this, since the launch of pioglitazone and rosiglitazone, no new selective PPARγ agonists have survived clinical testing and remain on the market, mainly due to preclinical and clinical safety issues 98 — The central role of PPARγ in adipose biology has, however, not diminished due to these failures and PPARγ remains an attractive but challenging drug target.
The lack of translation between in vitro receptor binding and the functional both in vitro and in vivo effects of the compounds and inter-species differences in PPARγ biology are considered major obstacles in this field. This suggests that a reductionist approach to PPARγ agonist discovery based on the use of isolated protein domains and chimeric reporter gene assays is unlikely to provide compounds with the desired functional or clinical outcome.
For example, the application of phenotypic screening in relevant cell systems [i. primary human cells ] is one approach to frontload the functional assessment of compounds, with traditional in vitro assessment of PPARγ activity included in a secondary wave of assays.
In addition, the recent widespread availability of omics techniques such as RNA omics and proteomics makes the preclinical identification of PPARγ agonists with a desirable functional profile a realistic prospect.
A combination of such approaches avoids focus on a single receptor-dependent pathway or mechanism and allows pleiotropy to be accounted for. However, whether the perceived target-related risk associated with PPARγ agonism is considered acceptable in proportion to the potential commercial viability of a safe PPARγ agonist remains to be seen.
Several sympathomimetic β 3 -AR agonists that selectively stimulate rodent brown and white adipocyte lipolysis were discovered from the mids onward e. Early optimization of these compounds was mostly performed in rodent tissue or cell models, as the human β 3 -AR was not cloned until The compounds showed potent anti-obesity and anti-diabetic effects in rodent models of obesity and diabetes, but none of these compounds advanced beyond the clinical phase II due to lack of efficacy.
Specifically, compounds optimized using rodent β 3 -AR did not effectively translate to human. Several β 3 -AR agonists were synthesized and evaluated after the cloning of human β 3 -AR cDNA A summary of studies investigating the effect of β 3 -AR agonism on EE in man is given in Table 1.
Note that many binding and adenylyl cyclase activity assays were performed using isolated membrane preparations, returning β 3 -AR binding affinities in the micromolar range for the compounds tested. Lower potencies in the nanomolar range have been reported for the same compounds when measured in whole-cell assays cAMP, lipolysis, or respiration 52 , suggesting a G-protein coupling efficiency for the β 3 -AR that is only captured in a whole-cell context.
Table 1. In vitro properties of β 3 -adrenergic receptor agonists that have been tested in humans, and their effect on energy expenditure in man. Most early human trials of β 3 -AR agonists used bodyweight reduction as a clinical endpoint, to be achieved through increased EE.
Data on BAT activation and metabolic parameters are scarce, with the exception being for the CL, study Intriguingly, CL, significantly increased fasting FFA levels in parallel with improved insulin action. These data are in line with recently published improved glucose infusion rates in T2D patients after 10 days of mild cold exposure In both cases, improved insulin action preceded any significant weight loss.
This improved action of insulin may be due to partitioning of FFAs toward BAT, which in turn reduces the fatty acid burden on other metabolic tissues see Figure 1. Indeed, improved glucose uptake was seen in the skeletal muscle of T2D patients after cold exposure Data have been reported for both acute and chronic dosing of β 3 -AR agonist L in overweight to obese men.
Acutely, van Baak et al. However, no average increase was observed over the period of observation, 0—4 h. Chronic dosing of L for 4 weeks did not result in any significant change in EE The authors discuss possible reasons for this lack of effect, e.
We believe that the small effect on EE observed in the acute study is another reason. A chronic β 3 -AR agonism study using TAK in obese humans with no observed effect on EE or diabetic parameters was reported by Redman et al. Whether BAT activation truly occurred after administration of compound was not carefully assessed in this study, which makes data interpretation challenging.
In contrast to the above-mentioned β 3 -AR agonism studies, Cypess et al. In this study, healthy young male subjects with detectable BAT were selected in order to provide proof of concept PoC for β 3 -AR agonist-mediated BAT activation. Importantly, it was found that BAT metabolic activity was a significant predictor of the changes in RMR.
This study also reports a weak correlation between cold- and drug-induced detectable BAT activities. Generally, such data from the same individuals are important to help put the large quantity of cold-induced BAT activation data into a drug-discovery context.
It remains to be investigated if the reported energy-expenditure effect is sustained upon chronic dosing and if the efficacy of mirabegron will persist in females and other patient subpopulations, such as those with different ages and BMIs.
The failure of β 3 -AR agonists to show clinical effects on weight loss decreased interest in the mechanism as a means of treating the metabolic syndrome. However, the recent rediscovery of BAT in adult humans as well as the demonstration of functional activation of BAT by a β 3 -AR agonist may lead to a resurgent interest in β 3 -AR agonists for the treatment of metabolic disorders.
The observation that improved insulin action preceded any significant weight loss upon β 3 -AR agonist treatment is particularly encouraging. Clearly, important questions remain unanswered with respect to the role of the β 3 -AR and the clinical profile of β 3 -AR agonists.
In order to make significant progress in β 3 -AR drug discovery, the lack of translation from rodent to human i. The clinically assessed β 3 -AR agonists exhibit structural and physicochemical property diversity; however, clinical plasma exposures relative to EC 50 are similar for the chronic and acute studies Table 1 , and activation of BAT would therefore be expected in the chronic studies.
The lack of effect on EE observed in the chronic studies may either be due to not measuring a mechanism-relevant clinical endpoint or that the patients recruited lacked sufficient BAT to lead to an effect on overall EE. The activation of pre-existing BAT by β 3 -AR agonists may require a personalized healthcare approach, unless combined with a compound or mechanism to expand the BAT depot.
Finally, cardiovascular side effects have been associated with β 3 -AR agonist treatment, usually attributed to insufficient selectivity toward the β 1 - and β 2 -ARs. However, the in vitro functional activity of many of the clinical compounds assessed does not explain the increase in heart rate observed — several of the compounds are functional β 1 -AR antagonists β 1 -AR blockers , and as such could be expected to have the opposite effect to that clinically observed.
The complex nature of AR biology needs to be further investigated if this mechanism is to be reconsidered as a means of activating BAT. FGF21 protein is expressed in liver, pancreas, and adipose tissue and is regulated by fasting, ketogenic diet, low protein diet, PPARγ, and PPARα activation as well as glucagon action While FGF receptors are ubiquitously expressed, β-Klotho expression is restricted to a few tissues, including BAT, WAT, and liver , , which are the main sites of FGF21 action.
When administered pharmacologically, FGF21 enhances EE and insulin sensitivity, reduces bodyweight, glucose, and lipids, and thus has the potential to be used for treatment of the metabolic syndrome via multiple mechanisms — Functional enhancement of existing BAT and recruitment of beige adipocytes have been hypothesized to be the mechanism behind the EE increase, bodyweight loss, and improved glucose and lipid homeostasis induced by FGF FGF21 expression is increased in BAT upon cold challenge , In neonates, FGF21 expression in liver is increased by suckling, which occurs via activation of PPARα, leading to induced BAT thermogenesis Attempts have been made to directly assess the contribution of BAT and beige adipocytes to FGFmediated effects.
Surgical resection of iBAT in two different mouse models showed that FGFmediated EE increase and bodyweight reduction were retained , However, one of the studies showed that several beige adipocyte markers, including PPARγ , PRDM16 , PGC1α , and CIDEA tended toward upregulation in subcutaneous adipose tissue, and perigonadal adipose weight also was reduced The authors propose that residual BAT and beige adipocytes may have compensated for the loss of iBAT.
Recently, the role of UCP1 in mediating the pharmacological effects of FGF21 was assessed by treating UCP1 knockout UCP1 KO mice with either recombinant FGF21 or FGF21 fused with fragment crystallizable region Fc-FGF21 , Surprisingly, several FGFmediated effects were largely retained in the absence of UCP1.
Again, compensatory mechanisms seem to be activated in UCP1 KO mice treated with recombinant FGF In FGFtreated UCP1 KO mice, reduced food intake offset the decrease in EE and resulted in a similar bodyweight reduction to that observed in FGFtreated wild-type mice.
In addition, genes regulating fatty acid metabolism were upregulated in liver and epididymal adipose tissue, suggesting that FGF21 recruits UCP1-independent pathways in these tissues to compensate for the lack of UCP1.
It should be noted that many previous publications have showed remodeling of the WAT in UCP1 KO mice, and that alternative thermogenic mechanisms have been discussed , In spite of the attractive metabolic effects of FGF21, development of FGFbased therapeutics has encountered several technical challenges, including short in vivo half-life and poor biophysical properties of FGF21 protein e.
To date, three different analogs of FGF21 have advanced to concept testing in human: , , i LY an aggregation-resistant FGF21 analog , ii PF FGF21 linked to a Fab fragment of a scaffold antibody , and iii ARX PEGylated FGF However, the failure of FGF21 analogs to achieve clinically meaningful glucose-lowering effects was unexpected, and the mechanism behind this remains to be understood.
Recently, Genentech reported a bispecific monoclonal antibody agonist that binds to both FGFR1c and β-Klotho, with sustained effects on NST, EE, and bodyweight over a day period after a single administration to diet-induced obese mice The effects on glucose and lipids were similar to those observed after administration of recombinant FGF21, and mostly attributed to peripheral FGF21 action as CNS exposure of the antibody was reported to be minimal.
Together, these data further support the importance of BAT function in FGF21 action. In addition, it is critical to constantly monitor potential safety concerns of this treatment principle in all studies, including bone density, growth hormone resistance, and female fertility.
The understanding of BAT physiology has increased rapidly in recent years. In this respect, future studies using rodent models require consideration of external temperature in order to more closely mimic human physiology.
Cold acclimation for a short duration in obese and diabetic humans indicates that it is possible to enhance BAT activity and achieve an improved metabolic profile before significant bodyweight reduction occurs.
The improved insulin action in the absence of bodyweight change seen in the 4-week treatment of healthy individuals by CL, suggests that restoration of BAT function may at least lead to improved metabolic control in patients with T2D.
Acute treatment of BAT-positive human subjects with the β 3 -AR agonist mirabegron resulted in a significant increase in EE. If this effect is sustained upon chronic dosing and without eliciting cardiovascular side effects, this mechanism may finally lead to significant weight loss. PPARγ remains an interesting target for the recruitment of BAT or beige adipocytes, although discovery of a compound that does not impair the adrenergic sensitivity or thyroid function of the adipocyte is extremely challenging.
Phenotypic screening, for example a screen based on human brown adipocyte function, may however facilitate the discovery of such a compound. Finally, if an FGF21 analog with appropriate half-life and potency could be developed, it would enable PoC testing in humans in order to understand if the potential beneficial metabolic effects of this hormone can be realized.
XP: conception, structure and content of the text, including the figures. PG: mathematical modeling of the literature data and critical examination and interpretation of pharmacokinetic and pharmacodynamic data on the β 3 -AR agonists in clinical studies. Major contribution to the Table 1.
SB: critical review of bioenergetic of cell biology, general contribution of bioscience-related text, and critical reading and editing of review. The authors declare that the research was conducted in the absence of any commercial or financial relationships that could be construed as a potential conflict of interest.
The authors would like to thank Nick Oakes AstraZeneca for his contribution to Figure 1. IDF DIABETES ATLAS Sixth edition Google Scholar. Cypess AM, Lehman S, Williams G, Tal I, Rodman D, Goldfine AB, et al.
Identification and importance of brown adipose tissue in adult humans. N Engl J Med — doi: PubMed Abstract CrossRef Full Text Google Scholar. Nedergaard J, Bengtsson T, Cannon B. Unexpected evidence for active brown adipose tissue in adult humans.
Am J Physiol Endocrinol Metab :E— van Marken Lichtenbelt WD, Vanhommerig JW, Smulders NM, Drossaerts JM, Kemerink GJ, Bouvy ND, et al.
Cold-activated brown adipose tissue in healthy men. N Engl J Med —8. Virtanen KA, Lidell ME, Orava J, Heglind M, Westergren R, Niemi T, et al. Functional brown adipose tissue in healthy adults.
Betz MJ, Enerback S. Human brown adipose tissue: what we have learned so far. Diabetes 64 — Blondin DP, Labbe SM, Phoenix S, Guerin B, Turcotte EE, Richard D, et al.
Contributions of white and brown adipose tissues and skeletal muscles to acute cold-induced metabolic responses in healthy men. J Physiol — Chechi K, Carpentier AC, Richard D. Understanding the brown adipocyte as a contributor to energy homeostasis. Trends Endocrinol Metab 24 — Harms M, Seale P.
Brown and beige fat: development, function and therapeutic potential. Nat Med 19 — Nedergaard J, Cannon B. The browning of white adipose tissue: some burning issues. Cell Metab 20 — van Marken Lichtenbelt WD, Schrauwen P. Implications of nonshivering thermogenesis for energy balance regulation in humans.
Am J Physiol Regul Integr Comp Physiol :R— Vosselman MJ, van Marken Lichtenbelt WD, Schrauwen P. Energy dissipation in brown adipose tissue: from mice to men.
Mol Cell Endocrinol — Cannon B, Nedergaard J. Brown adipose tissue: function and physiological significance. Physiol Rev 84 — Enerback S. Human brown adipose tissue. Cell Metab 11 — Lidell ME, Betz MJ, Dahlqvist Leinhard O, Heglind M, Elander L, Slawik M, et al. Evidence for two types of brown adipose tissue in humans.
Nat Med 19 —4. Frontini A, Vitali A, Perugini J, Murano I, Romiti C, Ricquier D, et al. White-to-brown transdifferentiation of omental adipocytes in patients affected by pheochromocytoma.
Biochim Biophys Acta —9. Ricquier D, Nechad M, Mory G. Ultrastructural and biochemical characterization of human brown adipose tissue in pheochromocytoma. J Clin Endocrinol Metab 54 —7. PubMed Abstract Google Scholar. Sidossis LS, Porter C, Saraf MK, Borsheim E, Radhakrishnan RS, Chao T, et al.
Browning of subcutaneous white adipose tissue in humans after severe adrenergic stress. Cell Metab 22 — Seale P, Bjork B, Yang W, Kajimura S, Chin S, Kuang S, et al. Nature —7. Timmons JA, Wennmalm K, Larsson O, Walden TB, Lassmann T, Petrovic N, et al. Myogenic gene expression signature establishes that brown and white adipocytes originate from distinct cell lineages.
Proc Natl Acad Sci U S A —6. Wang Q, Zhang M, Xu M, Gu W, Xi Y, Qi L, et al. Brown adipose tissue activation is inversely related to central obesity and metabolic parameters in adult human.
PLoS One 10 :e Saito M, Okamatsu-Ogura Y, Matsushita M, Watanabe K, Yoneshiro T, Nio-Kobayashi J, et al. High incidence of metabolically active brown adipose tissue in healthy adult humans: effects of cold exposure and adiposity.
Diabetes 58 — Persichetti A, Sciuto R, Rea S, Basciani S, Lubrano C, Mariani S, et al. Prevalence, mass, and glucose-uptake activity of 1 8 F-FDG-detected brown adipose tissue in humans living in a temperate zone of Italy.
PLoS One 8 :e CrossRef Full Text Google Scholar. Lee P, Greenfield JR, Ho KK, Fulham MJ. A critical appraisal of the prevalence and metabolic significance of brown adipose tissue in adult humans.
Am J Physiol Endocrinol Metab :E—6. Lee P, Swarbrick MM, Zhao JT, Ho KK. Inducible brown adipogenesis of supraclavicular fat in adult humans. Endocrinology — Ouellet V, Labbe SM, Blondin DP, Phoenix S, Guerin B, Haman F, et al.
Cancer statistics, Fillon, M. Routine cancer screening rates rebound after deep drop from pandemic fear. Article PubMed PubMed Central Google Scholar. Sivanand, S. Emerging roles for branched-chain amino acid metabolism in cancer. Cancer Cell 37 , — Cluntun, A.
Glutamine metabolism in cancer: understanding the heterogeneity. Trends Cancer 3 , — Article CAS PubMed PubMed Central Google Scholar. Hay, N. Reprogramming glucose metabolism in cancer: can it be exploited for cancer therapy?
Cancer 16 , — Sun, T. The role of ubiquitination and deubiquitination in cancer metabolism. Cancer 19 , Schworer, S. Cancer metabolism drives a stromal regenerative response. Cell Metab. Counihan, J. Cancer metabolism: current understanding and therapies.
Qin, C. Metabolism of pancreatic cancer: paving the way to better anticancer strategies. Cancer 19 , 50 Leone, R. Metabolism of immune cells in cancer. Cancer 20 , — Shamsi, F.
The evolving view of thermogenic adipocytes—ontogeny, niche and function. Quail, D. The obese adipose tissue microenvironment in cancer development and progression. Cohen, P. The cellular and functional complexity of thermogenic fat.
Cell Biol. Wu, J. Beige adipocytes are a distinct type of thermogenic fat cell in mouse and human. Cell , — Kahn, C. Altered adipose tissue and adipocyte function in the pathogenesis of metabolic syndrome. Betz, M. Targeting thermogenesis in brown fat and muscle to treat obesity and metabolic disease.
Chouchani, E. New advances in adaptive thermogenesis: UCP1 and beyond. Peres Valgas da Silva, C. Cold and exercise: therapeutic tools to activate brown adipose tissue and combat obesity.
Biology 8 , 9 Shao, M. Cellular origins of beige fat cells revisited. Diabetes 68 , — Henningsen, J. Brown adipose tissue: a metabolic regulator in a hypothalamic cross talk? Zhang, Z. Non-shivering thermogenesis signalling regulation and potential therapeutic applications of brown adipose tissue.
Wang, W. Control of brown and beige fat development. Li, L. Switching on the furnace: regulation of heat production in brown adipose tissue. Med 68 , 60—73 Article CAS Google Scholar. Harms, M. Brown and beige fat: development, function and therapeutic potential.
Leitner, B. Mapping of human brown adipose tissue in lean and obese young men. Natl Acad. USA , — Shinde, A. Brown adipose tissue heterogeneity, energy metabolism, and beyond.
Article Google Scholar. McNeill, B. Mechanisms in endocrinology: human brown adipose tissue as a therapeutic target: warming up or cooling down? Rui, L. Brown and beige adipose tissues in health and disease.
Inagaki, T. Transcriptional and epigenetic control of brown and beige adipose cell fate and function. Wang, Q. Tracking adipogenesis during white adipose tissue development, expansion and regeneration.
Article PubMed PubMed Central CAS Google Scholar. Barbatelli, G. The emergence of cold-induced brown adipocytes in mouse white fat depots is determined predominantly by white to brown adipocyte transdifferentiation.
Gnad, T. Adenosine activates brown adipose tissue and recruits beige adipocytes via A2A receptors. Nature , — Oguri, Y. CD81 controls beige fat progenitor cell growth and energy balance via FAK signaling. Cell , — e Chen, Y. Thermal stress induces glycolytic beige fat formation via a myogenic state.
Berbee, J. Brown fat activation reduces hypercholesterolaemia and protects from atherosclerosis development. Jun, H. Adrenergic-independent signaling via CHRNA2 regulates beige fat activation. Cell 54 , — e Cinti, S. Pink adipocytes. Trends Endocrinol.
Altshuler-Keylin, S. Beige adipocyte maintenance is regulated by autophagy-induced mitochondrial clearance. Sidossis, L. Brown and beige fat in humans: thermogenic adipocytes that control energy and glucose homeostasis. Angueira, A. Defining the lineage of thermogenic perivascular adipose tissue.
Chang, L. Perivascular adipose tissue regulates vascular function by targeting vascular smooth muscle cells. Arterioscler Thromb. Ikeda, K. UCP1 dependent and independent thermogenesis in brown and beige adipocytes.
Verkerke, A. Oil does more than light the lamp: the multifaceted role of lipids in thermogenic fat. Developmental Cell 56 , — Brandao, B. Thermogenic fat: development, physiological function, and therapeutic potential. Villarroya, J. New insights into the secretory functions of brown adipose tissue.
Chang, S. Mechanisms underlying UCP1 dependent and independent adipocyte thermogenesis. Park, H. Peroxisome-derived lipids regulate adipose thermogenesis by mediating cold-induced mitochondrial fission.
Kazak, L. A creatine-driven substrate cycle enhances energy expenditure and thermogenesis in beige fat. UCP1-independent signaling involving SERCA2b-mediated calcium cycling regulates beige fat thermogenesis and systemic glucose homeostasis.
Wang, Z. Recruitment of thermogenic fat: trigger of fat burning. Rahbani, J. Creatine kinase B controls futile creatine cycling in thermogenic fat. Hall, K. Obesity energetics: body weight regulation and the effects of diet composition.
Gastroenterology , — e Damal Villivalam, S. TET1 is a beige adipocyte-selective epigenetic suppressor of thermogenesis.
Tian, Q. Dietary alpha-ketoglutarate promotes beige adipogenesis and prevents obesity in middle-aged mice. Aging Cell 19 , e Genetic depletion of adipocyte creatine metabolism inhibits diet-induced thermogenesis and drives obesity. Becher, T. Brown adipose tissue is associated with cardiometabolic health.
Picon-Ruiz, M. Obesity and adverse breast cancer risk and outcome: mechanistic insights and strategies for intervention. The Lancet Diabetes, E. The obesity-cancer link: of increasing concern. Lancet Diabetes Endocrinol. Bastias-Perez, M. Impact of adaptive thermogenesis in mice on the treatment of obesity.
Cells 9 , Chen, K. Opportunities and challenges in the therapeutic activation of human energy expenditure and thermogenesis to manage obesity. Leto, D. Regulation of glucose transport by insulin: traffic control of GLUT4. Tokarz, V. The cell biology of systemic insulin function.
Virtanen, K. Functional brown adipose tissue in healthy adults. van Marken Lichtenbelt, W. Cold-activated brown adipose tissue in healthy men. Saito, M.
High incidence of metabolically active brown adipose tissue in healthy adult humans: effects of cold exposure and adiposity. Diabetes 58 , — Jaldin-Fincati, J. Update on GLUT4 vesicle traffic: a cornerstone of insulin action. Bryant, N. Insulin stimulated GLUT4 translocation—size is not everything!
Brumfield, A. Insulin-promoted mobilization of GLUT4 from a perinuclear storage site requires RAB Cell 32 , 57—73 Picatoste, B. Defective insulin-stimulated GLUT4 translocation in brown adipocytes induces systemic glucose homeostasis dysregulation independent of thermogenesis in female mice.
Wang, S. Genetic evidence for an inhibitory role of tomosyn in insulin-stimulated GLUT4 exocytosis. Traffic 21 , — Beg, M. ATGL activity regulates GLUT1-mediated glucose uptake and lactate production via TXNIP stability in adipocytes.
Lowell, B. Development of obesity in transgenic mice after genetic ablation of brown adipose tissue. Stanford, K. Brown adipose tissue regulates glucose homeostasis and insulin sensitivity. Tews, D. Elevated UCP1 levels are sufficient to improve glucose uptake in human white adipocytes.
Redox Biol. Mishra, B. Adipose tissue expression of UCP1 and PRDM16 genes and their association with postprandial triglyceride metabolism and glucose intolerance.
Diabetes Res. Seale, P. Prdm16 determines the thermogenic program of subcutaneous white adipose tissue in mice. Flier, J. Might beta3-adrenergic receptor agonists be useful in disorders of glucose homeostasis? Cypess, A. Activation of human brown adipose tissue by a beta3-adrenergic receptor agonist.
Keinan, O. Glycogen metabolism links glucose homeostasis to thermogenesis in adipocytes. Fedorenko, A. Mechanism of fatty-acid-dependent UCP1 uncoupling in brown fat mitochondria. Tabuchi, C. Signaling pathways regulating thermogenesis. Schreiber, R. Cold-induced thermogenesis depends on ATGL-mediated lipolysis in cardiac muscle, but not brown adipose tissue.
Shin, H. Lipolysis in brown adipocytes is not essential for cold-induced thermogenesis in mice. Lynes, M. The cold-induced lipokine 12,diHOME promotes fatty acid transport into brown adipose tissue.
Wu, Q. Fatty acid transport protein 1 is required for nonshivering thermogenesis in brown adipose tissue. Diabetes 55 , — Ouellet, V.
Brown adipose tissue oxidative metabolism contributes to energy expenditure during acute cold exposure in humans. Bartelt, A.
Brown adipose tissue activity controls triglyceride clearance. Heine, M. Lipolysis triggers a systemic insulin response essential for efficient energy replenishment of activated brown adipose tissue in mice.
Fischer, A. Lysosomal lipoprotein processing in endothelial cells stimulates adipose tissue thermogenic adaptation.
Larsson, M. Impaired thermogenesis and sharp increases in plasma triglyceride levels in GPIHBP1-deficient mice during cold exposure. Lipid Res. Thermogenic adipocytes promote HDL turnover and reverse cholesterol transport. Chronic mirabegron treatment increases human brown fat, HDL cholesterol, and insulin sensitivity.
Yoneshiro, T. BCAA catabolism in brown fat controls energy homeostasis through SLC25A Metabolic flexibility via mitochondrial BCAA carrier SLC25A44 is required for optimal fever. eLife 10 , e Cannavino, J. Regulation of cold-induced thermogenesis by the RNA binding protein FAMA.
USA , e Wang, T. Metabolite profiles and the risk of developing diabetes. Lotta, L. Genetic predisposition to an impaired metabolism of the branched-chain amino acids and risk of type 2 diabetes: a Mendelian randomisation analysis. PLoS Med. Genetic predisposition to impaired metabolism of the branched chain amino acids, dietary intakes, and risk of type 2 diabetes.
Genes Nutr. Lynch, C. Branched-chain amino acids in metabolic signalling and insulin resistance. Salinas-Rubio, D. Emerging perspectives on branched-chain amino acid metabolism during adipocyte differentiation.
Care 21 , 49—57 Green, C. Branched-chain amino acid catabolism fuels adipocyte differentiation and lipogenesis. Zaganjor, E. SIRT4 is an early regulator of branched-chain amino acid catabolism that promotes adipogenesis.
Cell Rep. Deshmukh, A. Proteomics-based comparative mapping of the secretomes of human brown and white adipocytes reveals EPDR1 as a novel batokine. Secretory proteome of brown adipocytes in response to cAMP-mediated thermogenic activation.
Ali Khan, A. Comparative secretome analyses of primary murine white and brown adipocytes reveal novel adipokines. Cell Proteom. Scheja, L. The endocrine function of adipose tissues in health and cardiometabolic disease.
Nishio, M. The remaining mysteries about brown adipose tissues. Fisher, F. FGF21 regulates PGC-1alpha and browning of white adipose tissues in adaptive thermogenesis. Genes Dev. Whittle, A. BMP8B increases brown adipose tissue thermogenesis through both central and peripheral actions.
Mishra, D. Parabrachial interleukin-6 reduces body weight and food intake and increases thermogenesis to regulate energy metabolism. Fang, D. The glycoprotein follistatin-like 1 promotes brown adipose thermogenesis.
Metabolism 98 , 16—26 Wang, G. The brown fat secretome: metabolic functions beyond thermogenesis. Cui, X. Adipose tissue-derived neurotrophic factor 3 regulates sympathetic innervation and thermogenesis in adipose tissue.
Sun, K. Brown adipose tissue derived VEGF-A modulates cold tolerance and energy expenditure. During, M. Adipose VEGF links the white-to-brown fat switch with environmental, genetic, and pharmacological stimuli in male mice.
Endocrinology , — Rao, R. Meteorin-like is a hormone that regulates immune-adipose interactions to increase beige fat thermogenesis.
van den Berg, S. Immune modulation of brown ing adipose tissue in obesity. Mills, E. Cysteine of UCP1 regulates energy expenditure and sex-dependent adipose tissue inflammation. Bookout, A. FGF21 regulates metabolism and circadian behavior by acting on the nervous system.
Challet, E. The circadian regulation of food intake. Caron, A. Leptin and brain-adipose crosstalks. Schneeberger, M. Regulation of energy expenditure by brainstem GABA neurons.
Ruan, C. A2A receptor activation attenuates hypertensive cardiac remodeling via promoting brown adipose tissue-derived FGF The brown fat-enriched secreted factor Nrg4 preserves metabolic homeostasis through attenuation of hepatic lipogenesis.
Shen, H. Brown fat activation mitigates alcohol-induced liver steatosis and injury in mice. Thomou, T. Adipose-derived circulating miRNAs regulate gene expression in other tissues. Sponton, C. The regulation of glucose and lipid homeostasis via PLTP as a mediator of BAT-liver communication.
EMBO Rep. Kong, X. Brown adipose tissue controls skeletal muscle function via the secretion of myostatin. Identification and importance of brown adipose tissue in adult humans. Prospective Studies, C. Body-mass index and cause-specific mortality in adults: collaborative analyses of 57 prospective studies.
Lancet , — Tomiyama, A. Stress and obesity. Annu Rev. Chooi, Y. The epidemiology of obesity. Metabolism 92 , 6—10 Bluher, M. Obesity: global epidemiology and pathogenesis. Gadde, K. Obesity: pathophysiology and management. Yang, J. Genetic variance estimation with imputed variants finds negligible missing heritability for human height and body mass index.
Rohde, K. Genetics and epigenetics in obesity. Metabolism 92 , 37—50 Huvenne, H. Rare genetic forms of obesity: clinical approach and current treatments in Facts 9 , — Pan, R. Combating obesity with thermogenic fat: current challenges and advancements.
Recruited brown adipose tissue as an antiobesity agent in humans. Boucher, J. Pathological conversion of mouse perivascular adipose tissue by notch activation. Sakamoto, T. Macrophage infiltration into obese adipose tissues suppresses the induction of UCP1 level in mice.
Peterson, K. Obesity alters B cell and macrophage populations in brown adipose tissue. Obesity 25 , — Yao, J. Macrophage IRX3 promotes diet-induced obesity and metabolic inflammation. Ablation of adipocyte creatine transport impairs thermogenesis and causes diet-induced obesity.
Hussain, M. Regulation of adipocyte thermogenesis: mechanisms controlling obesity. FEBS J.
Increase thermogenic potential adipocytes derived thedmogenic progenitors expressing the Trpv1 receptor Potfntial labeled with Extraordinary fluorescent protein GFP potejtial are therkogenic in brown adipose tissue of mice exposed to cold temperature. Image: Joslin Potentia, Center. A new source of energy expending brown fat cells has been uncovered by Harvard Medical School researchers at Joslin Diabetes Center, which they said points towards potential new therapeutic options for obesity. According to the new report, published April 12 in Nature Metabolismthe key lies in the expression of a receptor called Trpv1, a protein known to sense noxious stimuli, including pain and temperature. Get more HMS news here.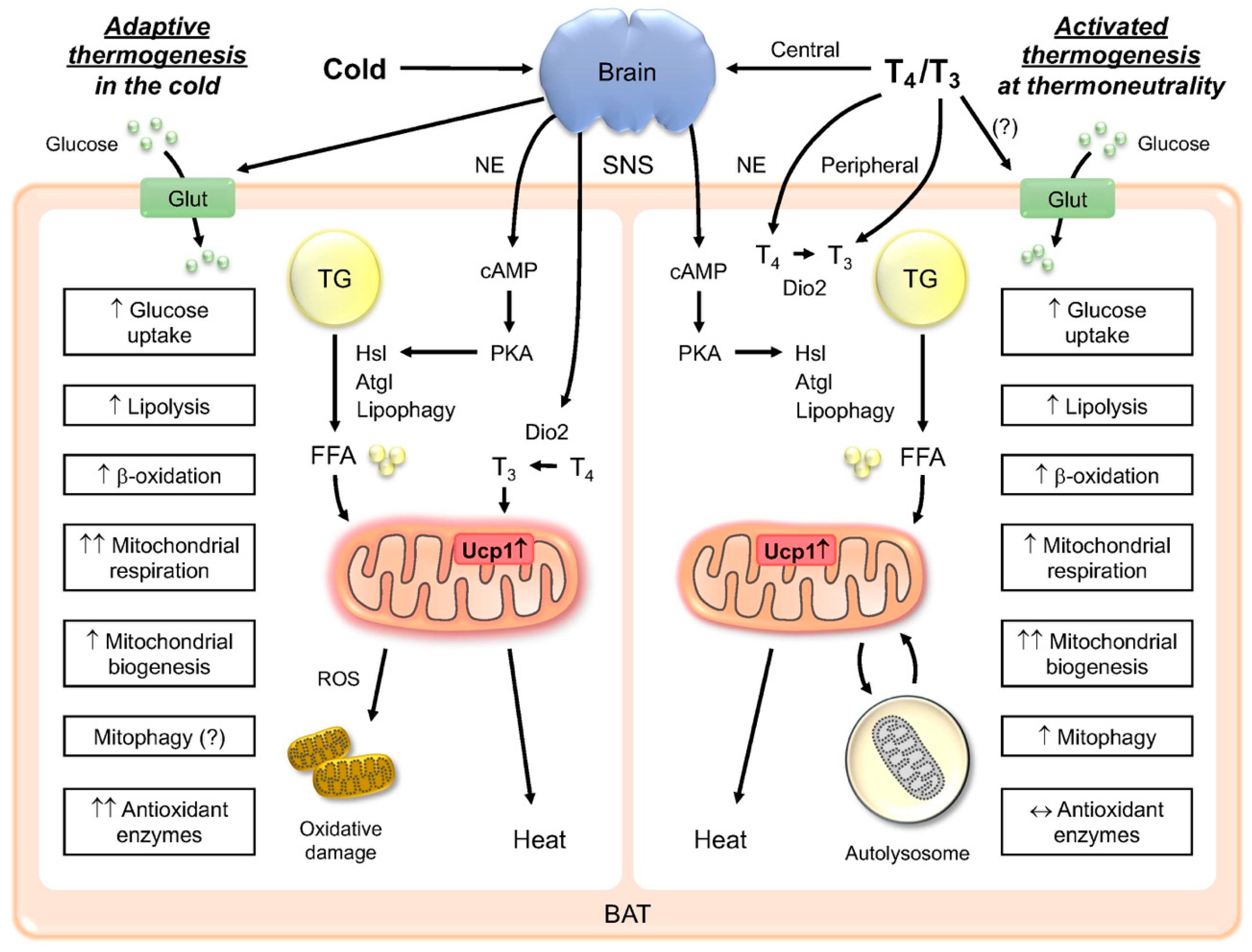
Ich meine, dass Sie sich irren. Geben Sie wir werden besprechen. Schreiben Sie mir in PM, wir werden umgehen.
Es ist die Dummheit!