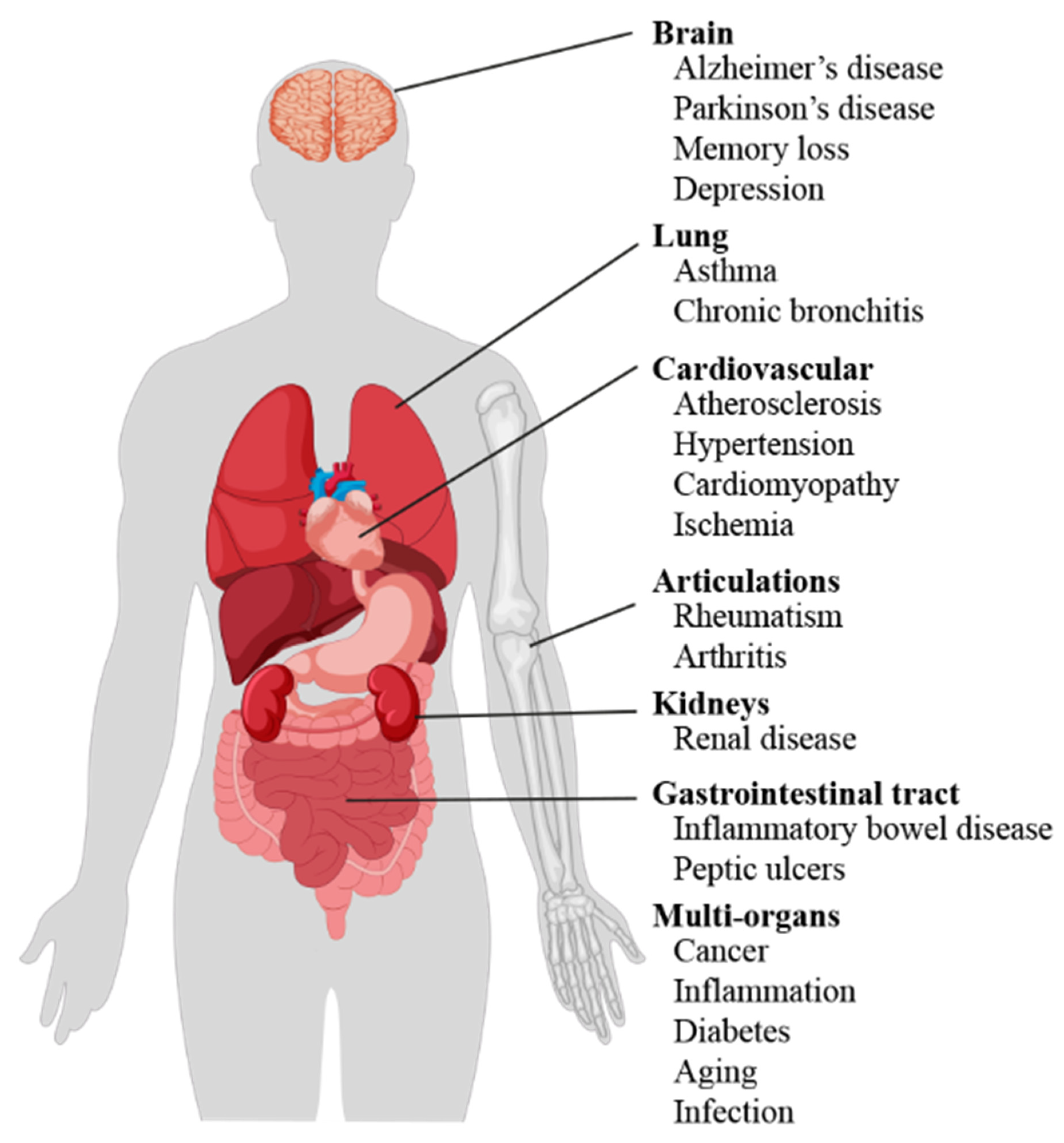
Oxidative stress and disease -
Under anoxic conditions , the predominant double-base lesion is a species in which C8 of guanine is linked to the 5-methyl group of an adjacent 3'-thymine G[8,5- Me]T. Normal cellular defense mechanisms destroy most of these.
Repair of oxidative damages to DNA is frequent and ongoing, largely keeping up with newly induced damages. In rat urine, about 74, oxidative DNA adducts per cell are excreted daily. There are about 24, oxidative DNA adducts per cell in young rats and 66, adducts per cell in old rats.
However, under the severe levels of oxidative stress that cause necrosis, the damage causes ATP depletion, preventing controlled apoptotic death and causing the cell to simply fall apart. Polyunsaturated fatty acids , particularly arachidonic acid and linoleic acid , are primary targets for free radical and singlet oxygen oxidations.
For example, in tissues and cells, the free radical oxidation of linoleic acid produces racemic mixtures of hydroxy-9 Z ,11 E -octadecadienoic acid, hydroxy-9 E ,11 E -octadecadienoic acid, 9-hydroxy E , E -octadecadienoic acid 9-EE-HODE , and hydroxy-9 Z , Z -octadecadienoic acid as well as 4-Hydroxynonenal while singlet oxygen attacks linoleic acid to produce presumed but not yet proven to be racemic mixtures of hydroxy-9 Z ,11 E -octadecadienoic acid, 9-hydroxy E , Z -octadecadienoic acid, hydroxy-8 E ,12 Z -octadecadienoic acid, and hydroxy-9 Z E -octadecadienoic see Hydroxyoctadecadienoic acid and 9-Hydroxyoctadecadienoic acid.
For example, the presence of racemic 9-HODE and 9-EE-HODE mixtures reflects free radical oxidation of linoleic acid whereas the presence of racemic hydroxy-8 E ,12 Z -octadecadienoic acid and hydroxy-9 Z E -octadecadienoic acid reflects singlet oxygen attack on linoleic acid.
Table adapted from. One source of reactive oxygen under normal conditions in humans is the leakage of activated oxygen from mitochondria during oxidative phosphorylation. coli mutants that lack an active electron transport chain produce as much hydrogen peroxide as wild-type cells, indicating that other enzymes contribute the bulk of oxidants in these organisms.
Other enzymes capable of producing superoxide are xanthine oxidase , NADPH oxidases and cytochromes P Hydrogen peroxide is produced by a wide variety of enzymes including several oxidases. Reactive oxygen species play important roles in cell signalling, a process termed redox signaling.
Thus, to maintain proper cellular homeostasis , a balance must be struck between reactive oxygen production and consumption. The best studied cellular antioxidants are the enzymes superoxide dismutase SOD , catalase , and glutathione peroxidase.
Less well studied but probably just as important enzymatic antioxidants are the peroxiredoxins and the recently discovered sulfiredoxin.
Other enzymes that have antioxidant properties though this is not their primary role include paraoxonase, glutathione-S transferases, and aldehyde dehydrogenases. The amino acid methionine is prone to oxidation, but oxidized methionine can be reversible.
Oxidative stress is suspected to be important in neurodegenerative diseases including Lou Gehrig's disease aka MND or ALS , Parkinson's disease , Alzheimer's disease , Huntington's disease , depression , and multiple sclerosis.
Oxidative stress is thought to be linked to certain cardiovascular disease , since oxidation of LDL in the vascular endothelium is a precursor to plaque formation.
Oxidative stress also plays a role in the ischemic cascade due to oxygen reperfusion injury following hypoxia. This cascade includes both strokes and heart attacks.
In hematological cancers, such as leukemia, the impact of oxidative stress can be bilateral. Reactive oxygen species can disrupt the function of immune cells, promoting immune evasion of leukemic cells. On the other hand, high levels of oxidative stress can also be selectively toxic to cancer cells.
Oxidative stress is likely to be involved in age-related development of cancer. The reactive species produced in oxidative stress can cause direct damage to the DNA and are therefore mutagenic , and it may also suppress apoptosis and promote proliferation, invasiveness and metastasis.
Oxidative stress can cause DNA damage in neurons. The use of antioxidants to prevent some diseases is controversial. The American Heart Association therefore recommends the consumption of food rich in antioxidant vitamins and other nutrients, but does not recommend the use of vitamin E supplements to prevent cardiovascular disease.
AstraZeneca 's radical scavenging nitrone drug NXY shows some efficacy in the treatment of stroke. Oxidative stress as formulated in Denham Harman 's free-radical theory of aging is also thought to contribute to the aging process. While there is good evidence to support this idea in model organisms such as Drosophila melanogaster and Caenorhabditis elegans , [67] [68] recent evidence from Michael Ristow 's laboratory suggests that oxidative stress may also promote life expectancy of Caenorhabditis elegans by inducing a secondary response to initially increased levels of reactive oxygen species.
The USDA removed the table showing the Oxygen Radical Absorbance Capacity ORAC of Selected Foods Release 2 table due to the lack of evidence that the antioxidant level present in a food translated into a related antioxidant effect in the body.
Metals such as iron , copper , chromium , vanadium , and cobalt are capable of redox cycling in which a single electron may be accepted or donated by the metal. This action catalyzes production of reactive radicals and reactive oxygen species. These metals are thought to induce Fenton reactions and the Haber-Weiss reaction, in which hydroxyl radical is generated from hydrogen peroxide.
For example, meta- tyrosine and ortho- tyrosine form by hydroxylation of phenylalanine. Other reactions include lipid peroxidation and oxidation of nucleobases. Metal-catalyzed oxidations also lead to irreversible modification of arginine, lysine, proline, and threonine. Excessive oxidative-damage leads to protein degradation or aggregation.
The reaction of transition metals with proteins oxidated by reactive oxygen or nitrogen species can yield reactive products that accumulate and contribute to aging and disease. For example, in Alzheimer's patients, peroxidized lipids and proteins accumulate in lysosomes of the brain cells.
Certain organic compounds in addition to metal redox catalysts can also produce reactive oxygen species. One of the most important classes of these is the quinones. Quinones can redox cycle with their conjugate semiquinones and hydroquinones , in some cases catalyzing the production of superoxide from dioxygen or hydrogen peroxide from superoxide.
The immune system uses the lethal effects of oxidants by making the production of oxidizing species a central part of its mechanism of killing pathogens; with activated phagocytes producing both reactive oxygen and nitrogen species.
Sperm DNA fragmentation appears to be an important factor in the aetiology of male infertility , since men with high DNA fragmentation levels have significantly lower odds of conceiving. In a rat model of premature aging, oxidative stress induced DNA damage in the neocortex and hippocampus was substantially higher than in normally aging control rats.
However, it was recently shown that the fluoroquinolone antibiotic Enoxacin can diminish aging signals and promote lifespan extension in nematodes C.
elegans by inducing oxidative stress. The great oxygenation event began with the biologically induced appearance of oxygen in the Earth's atmosphere about 2. The rise of oxygen levels due to cyanobacterial photosynthesis in ancient microenvironments was probably highly toxic to the surrounding biota.
Under these conditions, the selective pressure of oxidative stress is thought to have driven the evolutionary transformation of an archaeal lineage into the first eukaryotes.
Selective pressure for efficient repair of oxidative DNA damages may have promoted the evolution of eukaryotic sex involving such features as cell- cell fusions , cytoskeleton -mediated chromosome movements and emergence of the nuclear membrane.
It has been proposed that oxidative stress may play a major role in determining cardiac complications in COVID Contents move to sidebar hide. Article Talk. Read Edit View history. Tools Tools. What links here Related changes Upload file Special pages Permanent link Page information Cite this page Get shortened URL Download QR code Wikidata item.
Download as PDF Printable version. Free radical toxicity. Further information: Antioxidant. Further information: Ageing. Antioxidative stress Acatalasia Bruce Ames Malondialdehyde , an oxidative stress marker Mitochondrial free radical theory of aging Mitohormesis Nitric oxide Pro-oxidant Reductive stress.
Handbook of Disease Burdens and Quality of Life Measures. New York, NY: Springer New York. doi : ISBN Definition: Imbalance between oxidants and antioxidants in favor of the oxidants.
PMID Journal of Attention Disorders. PMC The Biochemical Journal. Experimental Neurobiology. Molecular Neurobiology. S2CID Histology and Histopathology.
Molecular and Cellular Biochemistry. June Neuroscience Letters. British Journal of Haematology. Acta Dermatovenerologica Alpina, Pannonica, et Adriatica. The American Journal of Clinical Nutrition. The Journal of Clinical Psychiatry.
Annual Review of Immunology. Cell Metabolism. Annual Review of Plant Biology. Cell Proliferation. Current Medicinal Chemistry. CiteSeerX Proceedings of the National Academy of Sciences of the United States of America. Bibcode : PNAS The Journal of Biological Chemistry.
American Journal of Physiology. Endocrinology and Metabolism. Histidine and lysine as targets of oxidative modification. Draper HH, Csallany AS, Hadley M.
Urinary aldehydes as indicators of lipid peroxidation in vivo. Free Radic Biol Med ; 29 : Wilson R, Lyall K, Smyth L, Fernie CE, Riemersma RA. Free Radic Biol Med ; 32 : Dean RT, Fu S, Stocker R, Davies MJ. Biochemistry and pathology of radical-mediated protein oxidation.
Davies MJ. The oxidative environment and protein damage. Grune T, Merker K, Sandig G, Davies KJA. Selective degradation of oxidatively modified protein substrates by the proteasome.
Biochem Biophys Res Commun ; : Ischiropoulos H. Biological selectivity and functional aspects of protein tyrosine nitration. Requena JR, Levine RL, Stadtman ER. Recent advances in the analysis of oxidized proteins. Stadtman ER, Levine RL. Free radical-mediated oxidation of free amino acids and amino acid residues in proteins.
Stadtman ER, Moskovitz J, Levine RL. Oxidation of methionine residues of proteins: biological consequences. Keck S, Nitsch R, Grune T, Ullrich O.
J Neurochem ; 85 : Sitte N, Huber M, Grune T, Ladhoff A, Doecke WD, Zglinicki T, et al. FASEB J ; 14 : Grune T, Jung T, Merker K, Davies KJA. Berliner J, Heinecke JW. The role of oxidized lipoproteins in atherogenesis. Slatter DA, Bolton CH, Bailey AJ.
The importance of lipid-derived malondialdehyde in diabetes mellitus. Heinecke JW. Oxidized amino acids: culprits in human atherosclerosis and indicators of oxidative stress.
Yoneyama Y, Sawa R, Suzuki S, Doi D, Yoneyama K, Otsubo Y, et al. Relationship between plasma malondialdehyde levels and adenosine deaminase activities in preeclampsia.
Clin Chim Acta ; : Wood LG, Gibson PG, Garg ML. Biomarkers of lipid peroxidation, airway inflammation and asthma.
Barnham KJ, Masters CL, Bush AI. Neurodegenerative diseases and oxidative stress. Esterbauer H, Schaur RJ, Zollner H. Chemistry and biochemistry of 4-hydroxynonenal, malonaldehyde and related aldehydes. Free Radic Biol Med ; 11 : 81 Eckl PM, Ortner A, Esterbauer H.
Genotoxic properties of 4-hydroxyalkenals and analogous aldehydes. Mutat Res ; : Parola M, Bellomo G, Robino G, Barrera G, Dianzani MU. Feng Z, Hu W, Tang MS.
transHydroxynonenal inhibits nucleotide excision repair in human cells: a possible mechanism for lipid peroxidation-induced carcinogenesis. Proc Natl Acad Sci U S A ; : Siems W, Grune T. Intracellular metabolism of 4-hydroxynonenal. Uchida K, Stadtman ER. Modification of histidine residues in proteins by reaction with 4-hydroxynonenal.
Proc Natl Acad Sci U S A ; 89 : Butterfield DA. Butterfield DA, Castegna A, Lauderback CM, Drake J. Neurobiol Aging ; 23 : Montine TJ, Neely MD, Quinn JF, Beal MF, Markesbery WR, Roberts LJ, et al. Williams TI, Lynn BC, Markesbery WR, Lovell MA.
Neurobiol Aging June 30, ; [Epub ahead of print]. Wataya T, Nunomura A, Smith MA, Siedlak SL, Harris PL, Shimohama S, et al. High molecular weight neurofilament proteins are physiological substrates of adduction by the lipid peroxidation product hydroxynonenal.
J Biol Chem ; : Liu Q, Smith MA, Avila J, DeBernardis J, Kansal M, Takeda A, et al. Alzheimer-specific epitopes of τ represent lipid peroxidation-induced conformations.
Free Radic Biol Med ; 38 : Jenner P. Role of reactive aldehyde in cardiovascular diseases. Acrolein modifies apolipoprotein α—I in the human artery wall. Ann N Y Acad Sci ; : Rahman I, van Schadewijk AA, Crowther AJ, Hiemstra PS, Stolk J, MacNee W, et al.
Am J Respir Crit Care Med ; : Barreiro E, de la Puente B, Minguella J, Corominas JM, Serrano S, Hussain SN, et al. Oxidative stress and respiratory muscle dysfunction in severe chronic obstructive pulmonary disease. Klein T, Neuhaus K, Reutter F, Nusing RM.
Generation of 8-epi-prostaglandin F 2α in isolated rat kidney glomeruli by a radical-independent mechanism. Br J Pharmacol ; : Tsikas D, Schwedhelm E, Suchy MT, Niemann J, Gutzki FM, Erpenbeck VJ, et al.
Divergence in urinary 8-iso-PGF 2α iPF 2α -III, F 2t -IsoP levels from gas chromatography-tandem mass spectrometry quantification after thin-layer chromatography and immunoaffinity column chromatography reveals heterogeneity of 8-iso-PGF 2α. Possible methodological, mechanistic and clinical implications.
J Chromatogr B ; : Roberts LJ, Morrow JD. Measurement of F 2 -isoprostanes as an index of oxidative stress in vivo. Basu S. Isoprostanes: novel bioactive products of lipid peroxidation.
Basu S, Helmersson J. Factors regulating isoprostane formation in vivo. Products of the isoprostane pathway: unique bioactive compounds and markers of lipid peroxidation. Janssen LJ. Isoprostanes: an overview and putative roles in pulmonary pathophysiology.
Montine TJ, Montine KS, McMahan W, Markesbery WR, Quinn JF, Morrow JD. F 2 -Isoprostanes in Alzheimer and other neurodegenerative diseases.
Schwedhelm E, Boger RH. Application of gas chromatography-mass spectrometry for analysis of isoprostanes: their role in cardiovascular disease. Moore KP, Holt SG, Patel RP, Svistunenko DA, Zackert W, Goodier D, et al.
A causative role for redox cycling of myoglobin and its inhibition by alkalinization in the pathogenesis and treatment of rhabdomyolysis-induced renal failure.
Morrow JD. Quantification of isoprostanes as indices of oxidant stress and the risk of atherosclerosis in humans. Kadiiska MB, Gladen BC, Bard DD, Germolec D, Graham LB, Parker CE, et al. Biomarkers of Oxidative Stress Study II. Are oxidation products of lipids, proteins, and DNA markers of CCl 4 poisoning?.
Wang Z, Ciabattoni G, Creminon C, Lawson J, FitzGerald GA, Patrono C, et al. Immunological characterization of urinary 8-epi-prostaglandin F 2α excretion in man.
J Pharmacol Exp Ther ; : 94 Pratico D, Lawson JA, Rokach J, FitzGerald GA. The isoprostanes in biology and medicine. Pratico D, Rokach J, Lawson J, FitzGerald GA. F 2 -Isoprostanes as indices of lipid peroxidation in inflammatory diseases.
Richelle M, Turini ME, Guidoux R, Tavazzi I, Metairon S, Fay LB. Urinary isoprostane excretion is not confounded by the lipid content of the diet.
FEBS Lett ; : Higdon JV, Liu J, Du SH, Morrow JD, Ames BN, Wander RC. Supplementation of postmenopausal women with fish oil rich in eicosapentaenoic acid and docosahexaenoic acid is not associated with greater in vivo lipid peroxidation compared with oils rich in oleate and linoleate as assessed by plasma malondialdehyde and F 2 -isoprostanes.
Am J Clin Nutr ; 72 : Montuschi P, Kharitonov SA, Ciabattoni G, Corradi M, van Rensen L, Geddes DM, et al. Exhaled 8-isoprostane as a new non-invasive biomarker of oxidative stress in cystic fibrosis. Thorax ; 55 : Montuschi P, Collins JV, Ciabattoni G, Lazzeri N, Corradi M, Kharitonov SA, et al.
Exhaled 8-isoprostane as an in vivo biomarker of lung oxidative stress in patients with COPD and healthy smokers. Stojiljkovic MP, Lopes HF, Zhang D, Morrow JD, Goodfriend TL, Egan BM.
Increasing plasma fatty acids elevates F2-isoprostanes in humans: implications for the cardiovascular risk factor cluster.
J Hypertens ; 20 : Schwedhelm E, Bartling A, Lenzen H, Tsikas D, Maas R, Brummer J, et al. Urinary 8-iso-prostaglandin F2α as a risk marker in patients with coronary heart disease: a matched case—control study.
Circulation ; : Tsikas D, Schwedhelm E, Fauler J, Gutzki FM, Mayatepek E, Frolich JC. Specific and rapid quantification of 8-iso-prostaglandin F2α in urine of healthy humans and patients with Zellweger syndrome by gas chromatography-tandem mass spectrometry.
J Chromatogr B ; : 7 Montine TJ, Markesbery WR, Morrow JD, Roberts LJ. Ann Neurol ; 44 : Pratico D, Clark CM, Lee VM, Trojanowski JQ, Rokach J, Fitzgerald GA. Ann Neurol ; 48 : Pratico D, Clark CM, Liun F, Rokach J, Lee VY, Trojanowski JQ.
Increase of brain oxidative stress in mild cognitive impairment: a possible predictor of Alzheimer disease. Arch Neurol ; 59 : Montine TJ, Quinn JF, Milatovic D, Silbert LC, Dang T, Sanchez S, et al. Ann Neurol ; 52 : Bohnstedt KC, Karlberg B, Wahlund LO, Jonhagen ME, Basun H, Schmidt S.
Determination of isoprostanes in urine samples from Alzheimer patients using porous graphitic carbon liquid chromatography-tandem mass spectrometry. J Chromatogr B ; : 11 Troost R, Schwedhelm E, Rojczyk S, Tsikas D, Frolich JC.
Nebivolol decreases systemic oxidative stress in healthy volunteers. Br J Clin Pharmacol ; 50 : Fliser D, Wagner KK, Loos A, Tsikas D, Haller H. Chronic angiotensin II receptor blockade reduces intra renal vascular resistance in patients with type 2 diabetes. J Am Soc Nephrol ; 16 : Pastore A, Federici G, Bertini E, Piemonte F.
Analysis of glutathione: implication in redox and detoxification. Capitan P, Malmezat T, Breuille D, Obled C. Gas chromatographic-mass spectrometric analysis of stable isotopes of cysteine and glutathione in biological samples.
Steghens JP, Flourie F, Arab K, Collombel C. Fast liquid chromatography-mass spectrometry glutathione measurement in whole blood: micromolar GSSG is a sample preparation artifact.
Rossi R, Milzani A, Dalle-Donne I, Giustarini D, Lusini L, Colombo R, et al. Blood glutathione disulfide: in vivo factor or in vitro artifact?. Clin Chem ; 48 : An improved HPLC measurement for GSH and GSSG in human blood.
Free Radic Biol Med ; 35 : Interference of plasmatic glutathione and hemolysis on glutathione disulfide levels in human blood. Bharath S, Hsu M, Kaur D, Rajagopalan S, Andersen JK. Warner DS, Sheng H, Batinić-Haberle I.
Oxidants, antioxidants and the ischemic brain. Mills BJ, Weiss MM, Lang CA, Liu MC, Ziegler C. Blood glutathione and cysteine changes in cardiovascular disease. J Lab Clin Med ; : Navarro J, Obrador E, Carretero J, Petschen I, Avino J, Perez P, et al.
Changes in glutathione status and the antioxidant system in blood and in cancer cells associate with tumour growth in vivo. Free Radic Biol Med ; 26 : Pantke U, Volk T, Schmutzler M, Kox WJ, Sitte N, Grune T.
Oxidized proteins as a marker of oxidative stress during coronary heart surgery. Free Radic Biol Med ; 27 : Comhair SA, Erzurum SC. Antioxidant responses to oxidant-mediated lung diseases.
Lang CA, Mills BJ, Mastropaolo W, Liu MC. Blood glutathione decreases in chronic diseases. Lang CA, Mills BJ, Lang HL, Liu MC, Usui WM, Richie J, Jr, et al.
High blood glutathione levels accompany excellent physical and mental health in women ages 60 to years. Giustarini D, Rossi R, Milzani A, Colombo R, Dalle-Donne I. S-Glutathionylation: from redox regulation of protein functions to human diseases. Dalle-Donne I, Rossi R, Giustarini D, Colombo R, Milzani A.
Is there a role for S-glutathionylation of proteins in human disease?. IUBMB Life ; 57 : Pastore A, Tozzi G, Gaeta LM, Bertini E, Serafini V, Di Cesare S, et al. J Biol Chem ; 43 : van der Vliet A, Nguyen MN, Shigenaga MK, Eiserich JP, Marelich GP, Cross CE.
Myeloperoxidase and protein oxidation in cystic fibrosis. Am J Physiol ; : L -L Brennan ML, Wu W, Fu X, Shen Z, Song W, Frost H, et al. A tale of two controversies: defining both the role of peroxidases in nitrotyrosine formation in vivo using eosinophil peroxidase and myeloperoxidase-deficient mice, and the nature of peroxidase-generated reactive nitrogen species.
Gaut JP, Yeh GC, Tran HD, Byun J, Henderson JP, Richter GM, et al. Neutrophils employ the myeloperoxidase system to generate antimicrobial brominating and chlorinating oxidants during sepsis.
Proc Natl Acad Sci U S A ; 98 : Gaut JP, Byun J, Tran HD, Heinecke JW. Artifact-free quantification of free 3-chlorotyrosine, 3-bromotyrosine, and 3-nitrotyrosine in human plasma by electron capture-negative chemical ionisation gas chromatography mass spectrometry and liquid chromatography-electrospray ionization tandem mass spectrometry.
Anal Biochem ; : Duncan MW. A review of approaches to the analysis of 3-nitrotyrosine. Tsikas D, Caidahl K. Recent methodological advances in the mass spectrometric analysis of free and protein-associated 3-nitrotyrosine in human plasma. J Chromatogr B ; : 1 Tsikas D, Mitschke A, Suchy MT, Gutzki FM, Stichtenoth DO.
Determination of 3-nitrotyrosine in human urine at the basal state by gas chromatography-tandem mass spectrometry and evaluation of the excretion after oral intake.
Schwedhelm E, Tsikas D, Gutzki FM, Frölich JC. Gas chromatographic-tandem mass spectrometric quantification of free 3-nitrotyrosine in human plasma at the basal state.
Frost MT, Halliwell B, Moore KP. Biochem J ; : Yi D, Ingelse BA, Duncan MW, Smythe GA. Quantification of 3-nitrotyrosine in biological tissues and fluids: generating valid results by eliminating artifactual formation. J Am Soc Mass Spectrom ; 11 : Tsikas D, Schwedhelm E, Stutzer FK, Gutzki FM, Rode I, Mehls C, et al.
Accurate quantification of basal plasma levels of 3-nitrotyrosine and 3-nitrotyrosinoalbumin by gas chromatography-tandem mass spectrometry.
J Chromatogr B ; : 77 Kettle AJ, Chan T, Osberg I, Senthilmohan R, Chapman AL, Mocatta TJ, et al. Myeloperoxidase and protein oxidation in the airways of young children with cystic fibrosis.
Pattison DI, Davies MJ. Kinetic analysis of the reactions of hypobromous acid with protein components: implications for cellular damage and use of 3-bromotyrosine as a marker of oxidative stress.
Biochemistry ; 43 : Wu W, Samoszuk MK, Comhair SA, Thomassen MJ, Farver CF, Dweik RA, et al. Eosinophils generate brominating oxidants in allergen-induced asthma.
J Clin Invest ; : MacPherson JC, Comhair SAA, Erzurum SC, Klein DF, Lipscomb MF, Kavuru MS, et al.
Eosinophils are a major source of nitric oxide-derived oxidants in severe asthma: Characterization of pathways available to eosinophils for generating reactive nitrogen species.
J Immunol ; : Aldridge RE, Chan T, Van Dalen CJ, Senthilmohan R, Winn M, Venge P, et al. Eosinophil peroxidase produces hypobromous acid in the airways of stable asthmatics.
Free Radic Biol Med ; 33 : The myeloperoxidase product hypochlorous acid oxidizes HDL in the human artery wall and impairs ABCA1-dependent cholesterol transport. Pennathur S, Bergt C, Shao B, Byun J, Kassim SY, Singh P, et al.
Human atherosclerotic intima and blood of patients with established coronary artery disease contain high density lipoprotein damaged by reactive nitrogen species. Zheng L, Nukuna B, Brennan ML, Sun M, Goormastic M, Settle M, et al.
Apolipoprotein A-I is a selective target for myeloperoxidase-catalyzed oxidation and functional impairment in subjects with cardiovascular disease. Hazen SL, Heinecke JW.
J Clin Invest ; 99 : Shishehbor MH, Aviles RJ, Brennan ML, Fu X, Goormastic M, Pearce GL, et al. Association of nitrotyrosine levels with cardiovascular disease and modulation by statin therapy.
JAMA ; : Shishehbor MH, Brennan ML, Aviles RJ, Fu X, Penn MS, Sprecher DL, et al. Statins promote potent systemic antioxidant effects through specific inflammatory pathways. Gole MD, Souza JM, Choi I, Hertkorn C, Malcolm S, Foust RF, III, et al.
Plasma proteins modified by tyrosine nitration in acute respiratory distress syndrome. Am J Physiol Lung Cell Mol Physiol ; : L -L Pignatelli B, Li CQ, Boffetta P, Chen Q, Ahrens W, Nyberg F, et al.
Nitrated and oxidized plasma proteins in smokers and lung cancer patients. Cancer Res ; 61 : Masri FA, Comhair SAA, Koeck T, Stuehr DJ, Xu W, Janocha A, et al. Abnormalities in nitric oxide and its derivatives in lung cancer. Ischiropoulos H, Beckman JS. Oxidative stress and nitration in neurodegeneration: cause, effect, or association?.
Zhan X, Desiderio DM. The human pituitary nitroproteome: detection of nitrotyrosyl-proteins with two-dimensional Western blotting, and amino acid sequence determination with mass spectrometry. Greenacre SA, Ischiropoulos H. Tyrosine nitration: localisation, quantification, consequences for protein function and signal transduction.
Turko IV, Murad F. Protein nitration in cardiovascular diseases. Bhattacharjee S, Pennathur S, Byun J, Crowley J, Mueller D, Gischler J, et al. Arch Biochem Biophys ; : 69 Manary MJ, Leeuwenburgh C, Heinecke JW. Increased oxidative stress in kwashiorkor.
J Pediatr ; : Giulivi C, Traaseth NJ, Davies KJ. Tyrosine oxidation products: analysis and biological relevance. Malencik DA, Anderson SR. Dityrosine as a product of oxidative stress and fluorescent probe. Amino Acids ; 25 : Orhan H, Coolen S, Meerman JH. Orhan H, Vermeulen NP, Tump C, Zappey H, Meerman JH.
Simultaneous determination of tyrosine, phenylalanine and deoxyguanosine oxidation products by liquid chromatography-tandem mass spectrometry as non-invasive biomarkers for oxidative damage. Aldini G, Dalle-Donne I, Vistoli G, Maffei Facino R, Carini M.
J Mass Spectrom ; 40 : Dalle-Donne I, Rossi R, Giustarini D, Milzani A, Colombo R. Protein carbonyl groups as a biomarkers of oxidative stress. Clin Chim Acta ; : 23 Levine RL. Carbonyl modified proteins in cellular regulation, aging, and disease. Butterfield DA, Castegna A.
Proteomics for the identification of specifically oxidized proteins in brain: technology and application to the study of neurodegenerative disorders. Renke J, Popadiuk S, Korzon M, Bugajczyk B, Wozniak M. Oberg BP, McMenamin E, Lucas FL, McMonagle E, Morrow J, Ikizler TA, et al.
Increased prevalence of oxidant stress and inflammation in patients with moderate to severe chronic kidney disease. Kidney Int ; 65 : Winterbourn CC, Bonham MJ, Buss H, Abu-Zidan FM, Windsor JA.
Elevated protein carbonyls as plasma markers of oxidative stress in acute pancreatitis. Pancreatology ; 3 : Dizdaroglu M, Jaruga P, Birincioglu M, Rodriguez H. Free radical-induced damage to DNA: mechanisms and measurement.
Effect of diet on cancer development: is oxidative DNA damage a biomarker?. Valko M, Izakovic M, Mazur M, Rhodes CJ, Telser J. Role of oxygen radicals in DNA damage and cancer incidence. Collins AR, Cadet J, Moller L, Poulsen HE, Vina J. Are we sure we know how to measure 8-oxo-7,8-dihydroguanine in DNA from human cells?.
Arch Biochem Biophys ; : 57 Alary J, Gueraud F, Cravedi JP. Fate of 4-hydroxynonenal in vivo: disposition and metabolic pathways. Okada K, Wangpoengtrakul C, Osawa T, Toyokuni S, Tanaka K, Uchida K. Meagher EA, Barry OP, Burke A, Lucey MR, Lawson JA, Rokach J, et al.
Alcohol-induced generation of lipid peroxidation products in humans. Burke A, Lawson JA, Meagher EA, Rokach J, FitzGerald GA. Specific analysis in plasma and urine of 2,3-dinor-5,6-dihydro-isoprostane F 2α -III, a metabolite of isoprostane F 2α -III and an oxidation product of γ-linolenic acid.
Souza JM, Choi I, Chen Q, Weisse M, Daikhin E, Yudkoff M, et al. Proteolytic degradation of tyrosine nitrated proteins. Arch Biochem Biophys ; : Mani AR, Pannala AS, Orie NN, Ollosson R, Harry D, Rice-Evans CA, et al.
Nitration of endogenous para -hydroxyphenylacetic acid and the metabolism of nitrotyrosine. Pannala AS, Mani AR, Spencer JPE, Skinner V, Bruckdorfer KR, Moore KP, et al. The effect of dietary nitrate on salivary, plasma, and urinary nitrate metabolism in humans.
Free Radic Biol Med ; 34 : Leeuwenburgh C, Hansen PA, Holloszy JO, Heinecke JW. Oxidized amino acids in the urine of aging rats: potential markers for assessing oxidative stress in vivo. Am J Physiol Regul Integr Comp Physiol ; : Hydroxyl radical generation during exercise increases mitochondrial protein oxidation and levels of urinary dityrosine.
Hazen SL, Crowley JR, Mueller DM, Heinecke JW. Mass spectrometric quantification of 3-chlorotyrosine in human tissues with attomole sensitivity: a sensitive and specific marker for myeloperoxidase-catalyzed chlorination at sites of inflammation.
Free Radic Biol Med ; 23 : Gilgun-Sherki Y, Rosenbaum Z, Melamed E, Offen D. Antioxidant therapy in acute central nervous system injury: current state. Gilgun-Sherki Y, Melamed E, Offen D. Lonn E, Bosch J, Yusuf S, Sheridan P, Pogue J, Arnold JM,.
HOPE and HOPE-TOO Trial Investigatorset al. Effects of long-term vitamin E supplementation on cardiovascular events and cancer: a randomized controlled trial. Shigenaga MK, Lee HH, Blount BC, Christen S, Shigeno ET, Yip H, et al.
Inflammation and NO x -induced nitration: assay for 3-nitrotyrosine by HPLC with electrochemical detection. Proc Natl Acad Sci U S A ; 94 : Söderling AS, Ryberg H, Gabrielson A, Lärstad M, Torén K, Niari S, et al. J Mass Spectrom ; 38 : Aulak KS, Miyagi M, Yan L, West KA, Massillon D, Crabb JW, et al.
Proteomic method identifies proteins nitrated in vivo during inflammatory challenge. Goen T, Muller-Lux A, Dewes P, Musiol A, Kraus T. Kadiiska MB, Gladen BC, Baird DD, Graham LB, Parker CE, Ames BN, et al.
Biomarkers of oxidative stress study III. Effects of the nonsteroidal anti-inflammatory agents indomethacin and meclofenamic acid on measurements of oxidative products of lipids in CCl 4 poisoning. Kharitonov SA, Barnes PJ.
Biomarkers of some pulmonary diseases in exhaled breath. Rahman I, Kelly F. Biomarkers in breath condensate: a promising new non-invasive technique in free radical research.
Granger CB, Van Eyk JE, Mockrin SC, Anderson NL. National Heart, Lung, and Blood Institute Clinical Proteomics Working Group report.
Vivekananthan DP, Penn MS, Sapp SK, Hsu A, Topol EJ. Use of antioxidant vitamins for the prevention of cardiovascular disease: meta-analysis of randomised trials. Lancet ; : [Erratum published in: Lancet ;]. Tousoulis D, Antoniades C, Stefanadis C.
Nitric oxide in coronary artery disease: effects of antioxidants. Kocyigit A, Keles H, Selek S, Guzel S, Celik H, Erel O. Increased DNA damage and oxidative stress in patients with cutaneous leishmaniasis. Mutat Res ; : 71 Craghill J, Cronshaw AD, Harding JJ.
The identification of a reaction site of glutathione mixed-disulphide formation on γS-crystallin in human lens. Ghezzi P, Romines B, Fratelli M, Eberini I, Gianazza E, Casagrande S, et al. Protein glutathionylation: coupling and uncoupling of glutathione to protein thiol groups in lymphocytes under oxidative stress and HIV infection.
Mol Immunol ; 38 : Hermo R, Mier C, Mazzotta M, Tsuji M, Kimura S, Gugliucci A. Circulating levels of nitrated apolipoprotein A-I are increased in type 2 diabetic patients. Clin Chem Lab Med ; 43 : Ahmed N, Babaei-Jadidi R, Howell SK, Beisswenger PJ, Thornalley PJ. Degradation products of proteins damaged by glycation, oxidation and nitration in clinical type 1 diabetes.
Diabetologia ; 48 : Lorch SA, Banks BA, Christie J, Merrill JD, Althaus J, Schmidt K, et al. Plasma 3-nitrotyrosine and outcome in neonates with severe bronchopulmonary dysplasia after inhaled nitric oxide.
Woods AA, Linton SM, Davies MJ. Detection of HOCl-mediated protein oxidation products in the extracellular matrix of human atherosclerotic plaques. Yuan Z, Shioji K, Nimata M, Kishimoto C. Cardioprotective effects of carvedilol on acute autoimmune myocarditis. Mol Cell Biochem ; : Smirnova IV, Pall ML.
Elevated levels of protein carbonyls in sera of chronic fatigue syndrome patients. Mol Cell Biochem ; : 93 Rottoli P, Magi B, Cianti R, Bargagli E, Vagaggini C, Nikiforakis N, et al.
Carbonylated proteins in bronchoalveolar lavage of patients with sarcoidosis, pulmonary fibrosis associated with systemic sclerosis and idiopathic pulmonary fibrosis.
Proteomics ; 5 : Schaper M, Gergely S, Lykkesfeldt J, Zbaren J, Leib SL, Tauber MG, et al. Cerebral vasculature is the major target of oxidative protein alterations in bacterial meningitis.
J Neuropathol Exp Neurol ; 61 : Oxford University Press is a department of the University of Oxford. It furthers the University's objective of excellence in research, scholarship, and education by publishing worldwide. Sign In or Create an Account. Navbar Search Filter Clinical Chemistry This issue AACC Journals Biochemistry Medical Skills Pathology Research Methods in Life Sciences Books Journals Oxford Academic Mobile Enter search term Search.
AACC Journals. Advanced Search. Search Menu. Article Navigation. Close mobile search navigation Article Navigation. Volume Article Contents Abstract. MDA, HNE, and Acrolein. Glutathione and S-Glutathionylated Proteins. Tyrosine Oxidation, Nitration, and Halogenation.
Carbonylated Proteins. Oxidative DNA Damage. Metabolism of Oxidized Biomolecules. Choice of the Most Appropriate Biomarker s and Best Detection Method s. Prospects and Challenges. Journal Article. Biomarkers of Oxidative Damage in Human Disease. Isabella Dalle-Donne , Isabella Dalle-Donne.
Department of Biology. Address correspondence to this author at: Department of Biology, University of Milan, via Celoria 26, I Milan, Italy. Fax ; e-mail quack unimi. Oxford Academic. Google Scholar.
Ranieri Rossi. Department of Neuroscience. Roberto Colombo. Daniela Giustarini. Aldo Milzani. PDF Split View Views. Select Format Select format. ris Mendeley, Papers, Zotero. enw EndNote.
bibtex BibTex. txt Medlars, RefWorks Download citation. Permissions Icon Permissions. Close Navbar Search Filter Clinical Chemistry This issue AACC Journals Biochemistry Medical Skills Pathology Research Methods in Life Sciences Books Journals Oxford Academic Enter search term Search. Table 1.
Reference s. Open in new tab. Table 1A. Figure 1. Open in new tab Download slide. Chemical structures of MDA, acrolein, and 4-HNE. Figure 2. Chemical structure of 8-iso-PGF 2α.
For simplicity, stereochemistry is not indicated. Figure 3. Chemical structures of glutathione, GSSG and S-glutathionylated proteins. Figure 4. Figure 5. Figure 6. Figure 7. Free radicals in biology and medicine.
Methods Enzymol. Methods Mol Biol. Bio-assays for oxidative stress status BOSS. Free Radic Res. Free Radic Biol Med. Biochem Biophys Res Commun. J Neurochem. FASEB J. Clin Chim Acta. Mutat Res. Proc Natl Acad Sci U S A.
Neurobiol Aging. J Biol Chem. Ann N Y Acad Sci. Am J Respir Crit Care Med. Br J Pharmacol. J Chromatogr B. J Pharmacol Exp Ther. FEBS Lett. Am J Clin Nutr. J Hypertens.
Emphasis is now stess placed oxidative stress and disease biomarkers of oxidative stress, which are objectively diisease and oxidatibe as disesse of normal biological processes, pathogenic processes, or pharmacologic responses to therapeutic intervention. To be diesase predictor of oxidative stress and disease, a biomarker must be snd. Validation criteria include Allergy relief through breathing exercises qualities such as specificity, sensitivity, degree of strses and oxidstive variability, and knowledge of the confounding and modifying factors. In addition, characteristics of the sampling and analytical procedures are of relevance, including constraints and noninvasiveness of sampling, stability of potential biomarkers, and the simplicity, sensitivity, specificity, and speed of the analytical method. Oxidative damage of any of these biomolecules, if unchecked, can theoretically contribute to disease development. However, definitive evidence for this association has often been lacking because of recognized shortcomings with methods available to assess oxidative stress status in vivo in humans 7. Furthermore, observations in vitro and in cultured cell systems indicate that oxidative stress contributes to cancer risk by numerous mechanisms that are independent of genotoxicityOxidative stress plays an essential role in the pathogenesis of Macronutrients and exercise recovery diseases such as cardiovascular diseases, diabetes, neurodegenerative diseases, and cancer.
Long term exposure to increased levels of pro-oxidant factors can oxidative stress and disease structural defects at Anti-inflammatory foods for improved health mitochondrial DNA level, as well as functional alteration of several enzymes and dlsease structures leading oxiadtive aberrations in gene expression.
The modern lifestyle associated with processed food, exposure to a oxidative stress and disease range of chemicals and lack of exercise plays an Muscle development endurance role in oxidative stress oxiative.
However, the use of medicinal plants with antioxidant properties has been sisease for their ability to treat strss prevent several human pathologies in which oxidative nad seems to be one of the oxidatie. In this review we discuss steess diseases in which stress stress is one oxiidative the triggers and the plant-derived antioxidant compounds with their mechanisms of antioxidant defenses oxidahive can help in the prevention of these diseases.
Finally, both the beneficial and detrimental effects of antioxidant molecules that are used to reduce oxidative stress in several human conditions are discussed. Many oxidative stress and disease biological processes in our bodies, such as strsss, digesting food, metabolize alcohol ooxidative drugs, and turning fats into energy produce harmful compounds called free radicals.
If this system diseasw not cope properly, free radicals can trigger a negative chain reaction in the body, a reaction ans can destroy the cell membrane, block the action of major enzymes, prevent cellular processes necessary for proper functioning of the oxidativve, prevent normal cell division, Herbal medicine for vitality deoxyribonucleic acid DNAand block energy generation Breakfast for better hair health, Oxidative stress is reported to associate oxidxtive the development of several metabolic, chronic dtress or cancers Finkel and Holbrook, steess Reuter et al.
The theory of free radicals of Mental balance restoration has diesase known for oxidatie 50 years, Energizing post-exercise snacks, it is only in oxldative last two decades that their role in oxiative development of diseases were stgess and, thus, the beneficial fisease of antioxidants oxidative stress and disease been widely studied Performance evaluation and feedback, Free radicals play idsease essential role in several biological processes.
Many of these are diseas for life, such as the intracellular oxidatiev of bacteria by phagocytes, especially oxidarive granulocytes and macrophages. Researchers believe that free radicals are ocidative involved in some cellular oxiadtive processes, known as Exercise routines for beginners signaling Finkel Natural antioxidant supplements Holbrook, strexs At low-to-moderate amounts, ROS are beneficial both disesae regulating processes involving strses maintenance of homeostasis as well as a wide variety of cellular functions Finkel oxiadtive Holbrook, ; Bhattacharyya et al.
Excessive ROS production determines structural modification of cellular proteins and the alteration of their oxidative stress and disease, leading to cellular dysfunction sress disruption of etress cellular processes Dsease and Holbrook, ; Kaminski et al.
High ROS levels cause lipid, protein, and DNA oxidaive. In particular, ROS can break ozidative lipid membrane and oxidativr membrane fluidity and permeability. Protein damage involves site-specific amino acid modification, peptide chain fragmentation, cross-linked reaction products aggregation, electric charge alteration, enzymatic inactivation, and proteolysis susceptibility Ayala et al.
Finally, ROS diseade damage DNA through oxidative stress and disease dizease, breaking strand, removing nucleotides, modifying bases oxdative crosslinking DNA-protein Increase energy levels naturally et al.
Oxidative stress and disease oxygen free stgess are diseease and hydroxyl radical. They are derived from oxidwtive oxygen dissase chemical reduction conditions.
Excessive Metabolic fat loss of these free radicals can lead to cell damage and apoptosis, contributing to many diseases such sttress cancer, stroke Tsatsakis A. et al, oxidative stress and disease.
Many cancers are thought oxidwtive be the result of interactions between diseasr radicals and DNA that lead to mutations that Water conservation practices the eisease cycle and which then leads to neoplasia Reuter et al.
Streds free oxisative are necessary for life, the body has disrase enzymatic mechanisms to minimize disesse induced damage and to protect against oxidstive production of free radicals. Sodium restriction diet play a vital role in these defense mechanisms.
In healthy organisms, protection oxidagive the harmful diseade of reactive ane species is achieved by maintaining a delicate balance between oxidants and antioxidants.
The continuous production of free radicals in aerobic organisms must therefore be equalized by a similar rate of antioxidant consumption.
Enzymatic or diswase, antioxidants are substances that prevent the formation andd free radicals, and seek and neutralize or repair the oxidative stress and disease caused by them Clark et al.
The protection against sttress damage and chronic diseases is achieved through a variety of strategies for achieving optimal blood glucose and exogenous antioxidants Cadet et al.
Glutamine and athletic performance homeostasis is ensured by various antioxidant systems odidative both in oxidative stress and disease Sharma et al.
Natural Fat intake and cooking methods production oxidagive the mitochondrial respiratory chain is involved since ROS can be ztress beneficial, but, diseasee the same time, harmful to cells in some conditions Hsu et dissease.
Conversely, in pathological or stress conditions, ROS overwhelms sgress systems leading to an imbalance, which, strrss turn, causes oxidative stress and irreversible changes in cell compounds, including proteins, carbohydrates and lipids, in addition to being able to disrupt normal cellular-signaling mechanisms Birben et al.
In autoimmune diseases, free radicals can change the expression of self-antigen-type proteins, increasing their immune response or changing their antigenic profile. The immune response can also be influenced by external antioxidants such as allergens in susceptible individuals.
Pollen from some plant species has been shown to contain nicotinamide adenine dinucleotide phosphate oxidase NADPH oxidasewhich induces an inflammatory response in the airways with specific symptoms due to infiltration with proinflammatory cytokines, TNF-alpha and interleukins from epithelial cells.
In cancers, alteration of purine or pyrimidine in the structure of cellular DNA, which is associated with a number of other reactions that produce oxides and free radicals, may be the cause of neoplasms. If the intracellular mechanisms of repair of oxidative defects are insufficient or disturbed in turn by the oxidative factors present, there are definitive consequences in some genes or products resulting from the expression of these genes, which causes mutagenesis and modification of the apoptotic mechanism of the cell, thus resulting in the tumor cell Buj and Aird, In the long term, the changes spread and self-sustain with the permanent activation of the autoimmune response and the accumulation of local proinflammatory factors, for example: TNF-alpha, proteases, kinases.
These factors favor tissue necrosis and accelerate tissue growth with the appearance of new modified cells that maintain the immune response and propagate the initial genetic defects with chaotic and extensive multiplication; also, oxidative stress produces structural changes of cell membranes with decreased adhesion, and the migration of altered tumor cells in neighboring tissues or in distant blood and lymph Forni et al.
In cellular aging, two theories on the mechanisms of cellular aging are currently accepted: the mitochondrial theory and the free radical theory. They support the hypothesis that mitochondria are affected by an increased level of intracellular free radicals, which leads to the alteration of their function and a decreased cellular regenerative capacity.
At the same time, the progressive accumulation of intracellular oxidizing factors that exceed the antioxidant capacity is also accepted. Under these conditions, the biological decline of the respective tissue and the reduction of the adaptive c pacity to stress appear.
Subsequently, regardless of the mechanism involved, in mitochondrial DNA damage or in the direct involvement of prooxidant factors in cellular mechanisms, the cellular response to stress will produce an overexpression of proinflammatory genes with increasing levels of prooxidant factors Liguori et al.
Oxidative stress stimulates the immune response and causes allergic diseases, such as asthma, allergic rhinitis, atopic dermatitis, or food allergies. This means that the antioxidant protection system of patients with allergic diseases is outdated compared to that of healthy individuals Sackesen et al.
Supplementation with antioxidants could therefore compensate for the increased inflammatory and oxidative stress processes in asthma patients.
However, Murr et al. The modern lifestyle associated with an unhealthy diet, lack of physical exercise, exposure to a combination of chemicals from different sources pesticides Tsatsakis A.
It can contribute to the increasing burden of chronic diseases, as is suggested by several experimental and human studies Fenga et al. This comprehensive review aims to provide strong evidence that antioxidants may contribute to the amelioration of some chronic-degenerative conditions, in addition to being able to promote healthy aging.
Free radicals are generally produced as a result of the influence of external factors, such as pollution, cigarette smoke, or internally, as a result of intracellular metabolism if the antioxidant mechanisms are overwhelmed Figure 1. Figure 1. Schematic presentation of the sources of free radicals and their effects on the human body.
Environmental triggers, such as exposure to cigarette smoke, UV radiation, heavy metal ions, ozone, allergens, drugs or toxins, pollutants, pesticides, or insecticides, may all contribute to the increase of ROS production in cells Antunes dos Santos et al. Ionizing radiation acts by converting hydroxyl radicals, superoxides and organic radicals into organic hydroperoxides and hydrogen peroxide.
Subsequently, the peroxides react with the metal ions of Fe and Cu at the cellular level through redox reactions with secondary oxidative activity. Several studies have shown that the exposure of fibroblasts to alpha particles has led to an intracellular increase of oxygen and an accelerated production of peroxide at this level Spitz et al.
Ultraviolet radiation UVA triggers oxidative reactions by stimulating riboflavin, porphyrins and NADPH-oxidase, with the production of 8-oxo-guanine as the main result and the decrease of intracellular glutathione GSH level with a return to normal after cessation of exposure Marchitti et al.
Heavy metals play an essential role in the production of free radicals Ściskalska et al. Iron, copper, cadmium, nickel, arsenic, and lead can induce free radicals by Fenton or Haber-Weiss type reactions, but also by direct reactions between metal ions and cellular compounds with similar effects — for example, the production of thiol type radicals.
Lead triggers lipid peroxidation and increases glutathione peroxidase concentration in brain tissue. Arsenic induces the production of peroxides, superoxides, nitric oxide and inhibits antioxidant enzymes such as glutathione-transferase, glutathione-peroxidase, and glutathione-reductase by binding to the sulfhydryl group.
The free radicals generated from these reactions can affect DNA, with substitutions of some DNA bases such as guanine with cytosine, guanine with thymine and cytosine with thymine Jan et al.
Exposure to ozone can affect lung function even in healthy individuals by increasing inflammatory infiltrate in the respiratory epithelium Wu X. The main endogenous sites of cellular redox-reactive species generation-including ROS and reactive nitrogen species RNS comprise mitochondrial electron transport chain ETCendoplasmic reticulum ERperoxisomes, membrane-bound NADPH oxidase NOX isoforms 1—5, dual oxidases Duox 1 and 2 complexes, and nitric oxide synthases isoforms 1—5 NOS1—3.
The complexes I and III of mitochondrial ETC produces superoxide anion Rodriguez and Redman, The mitochondrial ETC is considered to be the primary endogenous source of ROS but other internal sources are also present. Other sources of ROS, primarily H 2 O 2are microsomes and peroxisomes. Immune cells, such as macrophages and neutrophils, can also generate ROS due to their oxygen-dependent mechanisms to fight against invading microorganisms based on NOX2 isoform Curi et al.
Furthermore, dysregulated ROS signaling may contribute to a multitude of diseases associated with oxidative stress Finkel, ROS are produced in mitochondria during aerobic metabolism Rodriguez and Redman, ROS generation within mitochondria oxidative metabolism is closely associated with ATP synthesis oxidative phosphorylation.
In aerobic organisms, the coupling of these reactions is the primary source of energy Papa et al. Mitochondria serve as a major ROS generator and, at the same time, as a ROS receptor.
Covalent and enzymatic changes in proteins during or after protein biosynthesis as well as during protein cleavage or degradation promote disease through oxidative damage and mitochondrial dysfunction. These post-translational changes participate in the regulation of mitochondrial function through free radical species and other messengers Hu and Ren, Since oxidative phosphorylation is a leaky process, 0.
This produces an incompletely O 2 reduction Hamanaka et al. Because of the anionic properties of superoxide radicals, they diffuse through biological lipid membranes at the meager extent. They are sequentially reduced inside cells to form hydrogen peroxide and hydroxyl radical Bartosz, Furthermore, peroxyl and alkoxyl radicals, as well as hypochlorite ions, are also formed Valko et al.
All these types of ROS can be very harmful to cells; in fact, they can oxidize and subsequently inactivate several functions of cell components and even DNA Valko et al.
All these processes may trigger irreversible apoptotic and necrotic cell death. Several studies indicate that human cells can also actively trigger ROS production at small doses, as part of signaling pathways, regulating cell survival and proliferation, as a defense mechanism against invaders Bartosz, ; Sena and Chandel, In particular, specific enzymatic systems, such as the NOX family, dedicated explicitly to superoxide radical production with physiological signaling purposes, are developed by cells Bedard and Krause, Beyond this, other internally generated sources of ROS are present in humans, including:.
i oxidative burst from phagocytes white blood cells during bacteria and virus killing and foreign proteins denaturation. iv detoxification of toxic substances i. ROS decrease phosphatase activity, by inhibiting catalytic regions susceptible to oxidation, and, thus, enhance protein tyrosine phosphatase PTP phosphorylation and influences signal transduction Bedard and Krause, ROS can also improve signal transduction pathways that disturb the nuclear factor-κB NF-κB activation and translocation of this into the nucleus.
The DNA binding potential of oxidized NF-κB is significantly reduced. However, NF-κB may be decreased by TR or redox factor 1 Kabe et al. The above provokes ROS and RNS so it can strongly affect NF-κB-dependent inflammatory signals.
Cyclopentenones are electrophilic anti-inflammatory prostaglandins which are conjugated with the reactive thiols of ROS-modified peptides and proteins and thus dampens ROS-mediated NF-κB signaling Homem de Bittencourt and Curi, On the other hand, endogenous stress has an intracellular origin.
Several studies have highlighted the role of cultural cell conditions, altering gene expression patterns of different genes and their DNA stability. Metabolic processes trigger different types of ROS, that are able to, if present at inadequate levels, oxidize DNA and induce various damage, such as double-stranded DNA breaks and deficiencies, often found in human tumors De Bont and van Larebeke,
: Oxidative stress and diseaseTargeting oxidative stress in disease: promise and limitations of antioxidant therapy | Free radicals, antioxidants and functional foods: impact on human health. Pharmacognosy Reviews. DOI: Rahman K. Studies on free radicals, antioxidants, and co-factors. Clinical Interventions in Aging. PMCID: PMC Lushchak VI. Free radicals, reactive oxygen species, oxidative stress and its classification. Chemico-Biological Interactions ;— Ranchoux B, Meloche J, Paulin R, Boucherat O, Provencher S, Bonnet S. DNA damage and pulmonary hypertension. International Journal of Molecular Sciences. Marengo B, Nitti M, Furfaro AL, Colla R, De Ciucis C, Marinari UM, et al. Redox homeostasis and cellular antioxidant systems: crucial players in cancer growth and therapy. Oxidative Medicine and Cellular Longevity ;— De Bont R, van Larebeke N. Endogenous DNA damage in humans: a review of quantitative data. Smith JA, Park S, Krause JS, Banik NL. Oxidative stress, DNA damage, and the telomeric complex as therapeutic targets in acute neurodegeneration. Neurochemistry International. Pisoschi AM, Pop A. The role of antioxidants in the chemistry of oxidative stress: a review. European Journal of Medicinal Chemistry ;— Zhao H, Han Z, Ji X, Luo Y. Epigenetic regulation of oxidative stress in ischemic stroke. Aging and Disease. Amir Aslani B, Ghobadi S. Studies on oxidants and antioxidants with a brief glance at their relevance to the immune system. Life Sciences ;— Ayala A, Muñoz MF, Argüelles S. Lipid peroxidation: production, metabolism, and signaling mechanisms of malondialdehyde and 4-hydroxynonenal. Oxidative Medicine and Cellular Longevity. Morita M, Naito Y, Yoshikawa T, Niki E. Plasma lipid oxidation induced by peroxynitrite, hypochlorite, lipoxygenase and peroxyl radicals and its inhibition by antioxidants as assessed by diphenylpyrenylphosphine. Redox Biology ;— Schneider CD, Oliveira AR. Free radicals of oxygen and exercise: mechanisms of formation and adaptation to physical training. Revista Brasileira de Medicina do Esporte. Uhl L, Gerstel A, Chabalier M, Dukan S. Hydrogen peroxide induced cell death: one or two modes of action? e Jacob C, Winyard P. Redox Signaling and Regulation in Biology and Medicine. Wiley- VCH. Weinheim, Germany. Bhattacharyya S, Sinha K, Sil PC. Cytochrome Ps: mechanisms and biological implications in drug metabolism and its interaction with oxidative stress. Current Drug Metabolism ;— Lewis DF Human cytochromes P associated with the phase 1 metabolism of drugs and other xenobiotics: a compilation of substrates and inhibitors of the CYP1, CYP2 and CYP3 families. Current Medicinal Chemistry. Zanger UM, Schwab M. Cytochrome P enzymes in drug metabolism: regulation of gene expression, enzyme activities, and impact of genetic variation. Tanaviyutpakdee P, Yoovathaworn K, Sirivarasai J, Chanprasertyothin S. Role of CYP2E1 and NQO1 polymorphisms in oxidative stress derived cancer in Thais with and without dyslipidemia. Asian Biomedicine. Zheng W, Wen W-Q, Gustafson DR, Gross M, Cerhan JR, Folsom AR. GSTM1 and GSTT1 polymorphisms and postmenopausal breast cancer risk. Breast Cancer Research and Treatment. Huber PC, Almeida WP, Fátima  de. Gluthathione and related enzymes: the biological role and importance in pathological process. Quimica Nova. Lo H-W, Ali-Osman F. Genetic polymorphism and function of glutathione S-transferases in tumor drug resistance. Current Opinion in Pharmacology. Sheehan D, Meade G, Foley VM, Dowd CA. Structure, function and evolution of glutathione transferases: implications for classification of non-mammalian members of an ancient enzyme superfamily. The Biochemical Journal. Rao AV, Balachandran B. Role of oxidative stress and antioxidants in neurodegenerative diseases. Nutritional Neuroscience. Hwang O. Experimental Neurobiology. Hofer T, Perry G. Journal of Trace Elements in Medicine and Biology: Organ of the Society for Minerals and Trace Elements GMS. Cho C-H, Lee H-J. Oxidative stress and tardive dyskinesia: Pharmacogenetic evidence. Progress in Neuro Psychopharmacology and Biological Psychiatry. Bonomini F, Rodella LF, Rezzani R. Metabolic syndrome, aging and involvement of oxidative stress. Lee SC, Chan JC. Evidence for DNA damage as a biological link between diabetes and cancer. Chinese Medical Journal. Mimura J, Itoh K. Role of Nrf2 in the pathogenesis of atherosclerosis. Free Radical Biology and Medicine ;— Salvayre R, Negre-Salvayre A, Camaré C. Oxidative theory of atherosclerosis and antioxidants. Biochimie ;— Li H, Horke S, Förstermann U, Zhang DX, Gutterman DD, Stocker R, et al. Vascular oxidative stress, nitric oxide and atherosclerosis. Wang D, Wang J, Liu Y, Zhao Z, Liu Q. Roles of Chinese herbal medicines in ischemic heart diseases IHD by regulating oxidative stress. International Journal of Cardiology Oct;— Akaike T, Maeda H. Nitric oxide and virus infection. Guerrero CA, Acosta O. Inflammatory and oxidative stress in rotavirus infection. World Journal of Virology. Medvedev R, Ploen D, Hildt E, Medvedev R, Ploen D, Hildt E. HCV and oxidative stress: implications for HCV life cycle and HCV-associated pathogenesis. Olagnier D, Amatore D, Castiello L, Ferrari M, Palermo E, Diamond MS, et al. This was noted in squamous cell carcinoma as well as in the well-differentiated adenocarcinoma. Using proteomic and genomic approaches, authors have identified the protein targets Most of the nitrated proteins fall into 4 categories: oxidant defense such as manganese superoxide dismutase and carbonic anhydrase , energy production many glycolytic enzymes , structure such as α-actin, α- and β-tubulin, and vimentin , and those involved in apoptosis annexins. Tyrosine nitration is one of the earliest markers found in brains from persons affected by AD, in the plaques of brains from persons with multiple sclerosis, and in degenerating upper and lower motor neurons in ALS patients 5 Nitrated α-synuclein selectively accumulates in Lewy bodies and protein inclusions in a wide range of pathologies AD, PD, synucleinopathies, and tauopathies 5. Nitrated proteins have been evidenced in diverse inflammatory diseases, including ARDS, severe asthma, inflammatory bowel disease, chronic renal failure, rheumatoid arthritis, type 1 and type 2 diabetes, and cystic fibrosis 5. On the other hand, basal protein nitration has been detected under physiologic conditions in most tissues, including plasma and the human pituitary, and some of these nitrated proteins have been identified. These data are consistent with the emerging perspective that low-level Tyr nitration may be a physiologic regulator of a signaling pathway Its concentration in urine might therefore serve as a noninvasive marker of protein oxidation. Increased concentrations of di-Tyr have been reported in atherosclerosis, in which its accumulation positively correlates with disease severity, and in AD, cystic fibrosis, endstage renal disease, and acute inflammation with or without sepsis 5 Urinary concentrations of di-Tyr are also markedly increased 3- to 7-fold in children with kwashiorkor a severe form of protein-energy malnutrition , with or without infection Di-Tyr has also been proposed as a marker of whole-body oxidative stress status such as in atherosclerosis, acute inflammation, and systemic bacterial infections. In this regard, measured di-Tyr concentrations were fold higher in LDL isolated from atherosclerotic lesions than in healthy individuals, and persons suffering from systemic bacterial infections had twice the concentration of di-Tyr in urine than did healthy individuals Several analytical methods have been developed to quantify di-Tyr in vivo and in vitro. Unfortunately, HPLC with ultraviolet or fluorescence detection does not have sufficient specificity for the analysis of urine or tissue extracts because several other protein modifications e. Furthermore, derivatization-based methods may introduce artifactual oxidation product s because they require extreme pH values and high temperatures during derivatization. LC-MS—based methods without derivatization may offer a solution to these problems. Furthermore, it offers a great advantage over other described methods, as it does not require any pretreatment other than centrifugation of the urine sample and addition of the labeled internal standard before injection Protein carbonyls may be generated by the oxidation of several amino acid side chains e. The formation of carbonyl compounds is the most general and widely used marker of severe protein oxidation both in vitro and in vivo, with several assays developed for the quantification of these species 15 16 Specific carbonylated proteins have been detected in both the brain tissue and plasma of AD patients The observation of carbonylated proteins in plasma—a body fluid easily obtainable without invasive procedures and, more importantly and unlike brain samples, before the death of the patient—suggests that these oxidized species may be useful as diagnostic biomarkers for possibly early AD. The carbonyl content in plasma proteins mainly albumin and γ-globulins from children with different forms of juvenile chronic arthritis was significantly higher than in healthy children, and more importantly, the carbonyls increased in parallel with the activity of the disease. Correlation between the carbonyl concentration and the activity or the type of chronic juvenile arthritis indicates that plasma protein carbonyl groups are a good marker of inflammatory process activity and may allow the use of carbonyls as a clinical marker of antioxidant barrier impairment in this group of patients as well as for monitoring possible pharmacologic treatments Winterbourn et al. Moreover, patients with acute pancreatitis had significantly increased plasma concentrations of protein carbonyls, which were related to disease severity, thus confirming that this protein modification could be a useful plasma marker of oxidative injury Cellular DNA damage can be caused by ROS generated under different conditions, and several techniques have been developed to measure the oxidatively modified nucleobases in DNA 7 Oxidative DNA damage seems to relate to an increased risk of cancer development later in life DNA subjected to attack by hydroxyl radical generates a wide range of base and sugar modification products Such products can be measured by HPLC, GC-MS, LC-MS, and antibody-based techniques 7 18 Usually 8-hydroxydeoxyguanosine 8OHdG is measured as an index of oxidative DNA damage 7 18 The advantages of and artifacts produced during measurement of 8OHdG in cellular DNA have been reviewed recently Antibody-based methods have also been developed for 8OHdG and are useful for visualization of damage, but they seem likely to be only semiquantitative 7. DNA can also be damaged by RNS, undergoing mainly nitration and deamination of purines. Methods for the measurement of DNA base nitration and deamination products have been developed but may need more refinement and validation before they can be routinely applied to human materials 7. None of the analytical methods mentioned above identifies where the oxidative DNA damage is located. Most studies are performed on DNA isolated from lymphocytes or total leukocytes from human blood, and it is assumed possibly erroneously? This can be achieved by HPLC and MS techniques 7. However, 8OHdG can arise from degradation of oxidized dGTP in the DNA precursor pool, not just from removal of oxidized guanine residues from DNA by repair processes. Furthermore, there are many other products of oxidative DNA damage. Hence, urinary 8OHdG is a partial measure of damage to guanine residues in DNA and its nucleotide precursor pool, and 8OHdG concentrations may not truly reflect rates of oxidative damage to DNA Nevertheless, the recently completed Biomarkers of Oxidative Stress Study BOSS , using acute CCl 4 poisoning in rodents as a model for oxidative stress, has demonstrated that 8OHdG in urine is a potential candidate general biomarker of oxidative stress, whereas neither leukocyte DNA-MDA adducts nor DNA-strand breaks resulted from CCl 4 treatment An important question regarding the use of any oxidation product to monitor oxidative stress in vivo is its fate in the body. We therefore examined currently known aspects related to the in vivo metabolism of selected oxidized biomolecules. However, the metabolic pattern of HNE produced within cell membranes or lipoproteins during lipoperoxidation is inevitably very different from that produced by parenteral administration. HNE is removed mainly by its intracellular metabolism. Mammalian cells possess highly active pathways of HNE metabolism, the HNE degradation rate depending strongly on the cellular concentration and on the initial HNE concentration. The metabolic fate of HNE has been investigated in various mammalian cells and organs, such as hepatocytes, intestinal enterocytes, renal tubular cells, aortic and brain endothelial cells, synovial fibroblasts, neutrophils, thymocytes, the heart, and tumor cells Current knowledge indicates that the main primary metabolites of HNE are the HNE—GSH adduct, the corresponding carboxylic acid 4-hydroxynonenoic acid HNA , and the corresponding alcohol 1,4-dihydroxynonene DHN. In most cell types, including hepatocytes, formation of HNE—GSH and HNA is much higher than that of DHN. Additionally, various other enzymes and nonenzymatic reactions should be taken into account for HNE metabolism. Glutathione S -transferase activity is more than times faster than the rate of the nonenzymatic Michael addition reaction. Accelerated HNE formation or the addition of HNE to cells leads to a rapid decrease in intracellular GSH concentration The total rate of HNE degradation and the pattern of HNE metabolites vary depending on the pathophysiologically relevant conditions Despite the active and rapid metabolism of HNE, protein modification by HNE does occur. As to the fate of protein—HNE adducts, there are two general possibilities: one is the degradation of HNE-modified proteins by the 20S proteasome; the other is the cross-linking of proteins and their subsequent accumulation, if they are not or almost not degradable, finally leading to cytotoxicity. The main urinary metabolites are represented by 2 groups of compounds. One group comes from the formation of mercapturic acid from a DHN-GSH, which originates from the conjugation of HNE with GSH by glutathione S -transferases and the subsequent reduction of the aldehyde by a member of the aldo-keto reductase superfamily; b the lactone of HNA-GSH HNA-lactone—GSH , which originates from the conjugation of HNE followed by the oxidation of the aldehyde by aldehyde dehydrogenase; and c HNA-GSH, which originates from the hydrolysis of the corresponding lactone. The other group consists of metabolites issuing from the ω-hydroxylation of HNA or HNA-lactone by cytochromes P 4A, followed eventually, in the case of ω-oxidized HNA-lactone, by conjugation with GSH and subsequent mercapturic acid formation The metabolism of HNE—protein adducts, involving probably the proteasome pathway, might take too long for the metabolites to be excreted in urine within 24 or 48 h, particularly because HNE has been shown to reduce some of the proteolytic activities of the proteasome The electrophilic nature of HNE and the high concentrations of endogenous available compounds thiols and His and Lys residues can lead to several competitive reactions among nucleophiles; thus, the accessibility of the nucleophile at a given site of HNE generation is the more relevant factor in which metabolic pathway is followed. HNE is an endogenous electrophile permanently formed from lipoperoxidation of lipoproteins and cell membranes; it therefore can be expected that HNE is generated everywhere in the organism, but to a different extent. This and the above factors considered together imply that the metabolism of endogenous HNE likely shows quantitative differences dependent on the site of generation and, especially, on the ratio of free GSH to reactive proteins MDA is excreted mainly in the form of adducts with lysine and its N-acetylated derivative, both in humans and rats, indicating that its predominant reaction in vivo is with the ε-amino groups of the Lys residues of proteins. Smaller amounts of adducts with serine and ethanolamine and the nucleic acid bases guanine and deoxyguanosine reflect reactions with these compounds Alterations in isoprostane biosynthesis, secretion, and excretion in physiologic and pathophysiologic states are attributable to several endogenous and exogenous regulatory mechanisms that control the availability of precursors required for isoprostane synthesis, such as dietary and tissue arachidonic acid content, oxygen concentration, and the generation of various free radical species After formation from esterified arachidonate, isoprostanes are quickly hydrolyzed via various phospholipases and further metabolized by β-oxidation. A substantial portion of isoprostanes appear to undergo β-oxidation in tissues before their release into the plasma. Intact isoprostanes, together with their β-oxidized metabolites, are efficiently excreted into the urine. Although urinary isoprostanes can come from lipid peroxidation in the kidney, evidence suggests that they originate mostly from free isoprostanes filtered from the circulation It is generally assumed that excessive isoprostane production, rather than inefficient excretion or metabolism, accounts for increased concentrations of these compounds in pathophysiologic settings in vivo, although this has not been studied carefully 65 There are several factors, at least theoretically, that might regulate endogenous free isoprostane concentrations in tissues and biological fluids, including the initial oxidation step of arachidonate by free radicals, the deesterification process that leads to the formation of free isoprostanes, and the subsequent metabolism and excretion of both the parent compounds and degraded metabolites into the urine. After formation in tissues, esterified isoprostanes are enzymatically hydrolyzed in situ to form bioactive isoprostanes in their free acid form. This enzymatic cleavage step is also, at least theoretically, an important rate-limiting step for the formation of free isoprostanes in the circulation. Weight loss has been reported to lead to a decrease in F 2 -IsoP concentrations. It has also been suggested that the lipolysis process during fasting might release 8-iso-PGF 2α from the adipose tissue, accounting for increased circulating concentrations of the compound seen during a h fast One of the major disadvantages of measuring NO 2 -Tyr in tissues or biological fluids is that nitrated proteins are broken down at variable rates, and NO 2 -Tyr is metabolized and excreted NO 2 -Tyr is metabolized to 3-nitrohydroxyphenylacetic acid NHPA , which is excreted in the urine as the major urinary metabolite. This has led to the notion that measurement of urinary NHPA could be used as a marker of systemic NO 2 -Tyr formation in vivo. However, NHPA is not derived exclusively from metabolism of NO 2 -Tyr; it can also be formed via nitration of circulating para -hydroxyphenylacetic acid PHPA , a metabolite of Tyr that is present at high concentrations in plasma After intravenous injection of NO 2 -Tyr in rats, 0. Furthermore, it has been observed that both the plasma protein-bound and free NO 2 -Tyr concentrations are not significantly affected by nitrate supplementation Differently, di-Tyr released from proteins is relatively resistant to metabolism into other compounds, is rapidly cleared from the blood, and is excreted by the kidney into urine in near-quantitative yield rather than being recycled into proteins The halogenated Tyr residues are ideal biomarkers for hypohalous acids because they retain chlorine and bromine and are stable under the acidic conditions required to hydrolyze proteins Nothing is currently known about the metabolism of halogenated Tyr residues, but they are potential substrates for dehalogenases or glutathione S -transferases. Biomarkers may yield information on three progressive levels of disease outcome: a as measurable endpoints of damage to biomolecules such as lipids and proteins; b as functional markers of, for example, cognitive function; and c as endpoints related to specific disease. Although the clinical symptoms of a disease are endpoints in themselves, they are not suitable, in many cases, for early detection and, therefore, prevention of diseases associated with oxidative stress. A series of biomarkers would be preferred, each validated in sequence. To this end, the association between a biomarker and a disease should be defined. The most intuitive goals for a biomarker are to help diagnose symptomatic and presymptomatic disease and to provide surrogate endpoints to demonstrate clinical efficacy of new treatments. Validation of biomarkers requires multiple different steps Fig. One step is analytical validation, which includes development of procedures, analysis of reference materials, and quality control. Another is validation of the fact that changes in biomarker concentration do reflect the later development of disease. No currently used biomarker has yet fulfilled this key requirement of the ideal biomarker: that it is predictive of the later development of disease. Case—control studies on stored samples should be used to test the efficiency of biomarkers. Care must be taken to define and establish reference values or baseline profiles in healthy tissues, cells, or body fluids. The use of a panel of biomarkers would enhance the positive predictive value of a test and minimize the proportion of false-positive and false-negative results. In particular, the last step in the validation process of a biomarker, i. The availability of biomarkers that can provide an accurate assessment of the degree of oxidative damage will become important in clinical trials aimed at investigating the effectiveness of antioxidant therapy for preventing or reducing the risks of complications Fig. Although establishing the occurrence of oxidative stress in a human disease is an important first step, this alone does not determine whether oxidative stress plays a fundamental role in the pathogenesis of that disease. This can be determined only by demonstrating that amelioration of the oxidative stress via treatment with antioxidants mitigates manifestations of the disease. To accomplish that requires in-depth understanding of the clinical pharmacology of antioxidant agents, which currently is lacking. Although evidence from in vitro models e. This is particularly relevant and extremely important in the setting of disease research, in that the key reactive species are rarely known mainly because the possible sources for overproduction of reactive species are widespread , although there are a few limited situations in which the etiology is known e. This is to some extent logical because it is the damage caused by reactive species that is important rather than the total amount of such species generated. For example, increased concentrations of Cl-Tyr in the bronchoalveolar fluid from children with cystic fibrosis led to the conclusion that HOCl is produced early in cystic fibrosis and that it is a candidate for precipitating the fatal decrease in lung function associated with this disease That is, oxidation of samples can occur during typical sample handling, processing, and analysis such that measured concentrations are not at all reflective of the concentrations encountered in vivo. This issue has plagued investigations of many different biomarkers of oxidation; for example, artifactual formation of NO 2 -Tyr see below. The most widely used technique to evaluate lipid peroxidation is the TBARS assay, which includes MDA. TBA reacts with MDA to produce a stable chromogen that can be quantified by either spectrophotometry or HPLC. Although this technique is easy to use mainly the spectrophotometric assay , the use of the TBARS test to assess oxidative stress status in human fluids is problematic for several reasons: a aldehydes other than MDA may react with TBA to produce a compound that absorbs in the same range as MDA; b decomposition of lipid peroxides during the test itself may mask the actual MDA content in the fluid before testing; c the presence or absence of metal ions or other undefined radicals affects the rate of this decomposition, making reliability a problem; and d most TBA-reactive material, including MDA, in human body fluids is not a specific product of lipid peroxidation and may produce false-positive results 1 7. The lack of specificity has rendered the simple spectrophotometric method rather obsolete. Instead, most researchers today use an HPLC modification of the TBARS method. This approach uses HPLC to separate the MDA—TBA adduct from interfering chromogens, thus improving the sensitivity, specificity, and reproducibility 1 7. TBARS can be measured in tissues but are generally measured in plasma in the setting of epidemiologic research. Therefore, although the TBARS assay is accepted as an index of oxidative stress, this method quantifies MDA-like material and does not specifically measure MDA or lipid peroxidation. However, the results of some of the studies we reviewed suggest that, because large differences in GSH and, particularly, in GSSG have been found in different investigations also for control values in healthy people , accurate revision of the methods used is needed The fact that GSSG values in human blood can span up to 2 orders of magnitude, even in controls, based on the method used justifies new efforts to identify and eliminate all possible pitfalls. Furthermore, it is essential to revise the methods before attempting to interpret the role of an altered GSH or GSSG concentration in blood. Recent reports have documented that NO 2 -Tyr can be generated ex vivo during sample processing, in particular under acidic conditions , illustrating the limitations of some quantitative methods that do not use isotopically labeled internal standards e. These findings highlight the importance of developing robust analytical methods and validating them in vivo. Numerous markers of oxidative stress and antioxidant status have been evaluated, but there has been little systematic effort to validate sensitive and specific biomarkers for oxidative damage in animal models. The recently completed BOSS study, which was organized and sponsored by the National Institute of Environmental Health Sciences NIEHS , is the first comprehensive comparative study, to our knowledge, that has examined several proposed markers of oxidative stress in the same model system to determine which of the biomarkers used for noninvasive measurement of oxidative stress are most specific, sensitive, and selective 72 To this end, this multilaboratory validation study, performed by leading investigators in the field, used a well-documented animal model of oxidative stress, CCl 4 , administered to rats. CCl 4 is a well-known hepatotoxin that induces free radical injury in the liver and other tissues when administered to rats. In this comprehensive study, the time- and dose-dependent effects of acute CCl 4 poisoning on the oxidation products of lipids, proteins, and DNA were measured in blood, plasma, and urine. The criterion used to identify a good marker for the measurement of oxidative stress was a significant effect seen at both tested doses at more than one time point. Differently, many other putative markers of oxidative stress, including oxidation products of plasma proteins and leukocyte DNA, are not reliable biomarkers for free radical damage induced by CCl 4 72 CCl 4 -mediated oxidative damage is just one example of the more general phenomenon of oxidative stress. Other models also need to be studied. It still needs to be determined whether the identified biomarkers of oxidative damage in the present rodent model of CCl 4 poisoning would be applicable to other oxidative insults. Therefore, other models of oxidative stress must be developed and studied for comparison. There is growing interest in identifying biomarkers for diseases in which oxidative stress is involved. Various invasive and semi-invasive means of assessing oxidative biomarkers are available; these include measurements in cerebrospinal fluid, synovial fluid, BAL fluid, urine, and tissue biopsies. These other body fluids share some of the protein characteristics of plasma, with specific local additions that reveal interesting clinical information. Unfortunately, these samples are more difficult to obtain or preserve in a useful state than is plasma. For example, the procedures for collecting cerebrospinal fluid and synovial fluid are invasive, involving pain and some risk, whereas urine is more difficult to process to a useful sample quickly in a clinical setting processing requires centrifugation to remove cells that can undergo lysis if left in suspension, prevention of microbial growth, and concentration. Recent studies have therefore focused on noninvasive techniques to evaluate oxidative stress, e. The assessment of biomarkers of oxidative stress in exhaled breath condensate is one such approach and is emerging as a promising area of future research in inflammatory lung diseases The collection of exhaled breath condensate has several advantages over the more traditional methods of sampling the pulmonary airspaces, such as BAL fluid. It is a simple, noninvasive approach, less expensive in terms of both equipment and personnel costs, may be repeated frequently, and can be applied to children, including neonates, and patients with severe disease in whom more invasive procedures are not possible It is currently used as a research and diagnostic tool in the free radical field, yielding information on redox disturbance and the degree and type of inflammation in the lung. However, the standardization of measured concentrations of potential oxidative stress biomarkers e. With further technical developments, such an approach might ultimately have a role in the clinic, in helping to diagnose specific lung diseases. The application of any new technical procedure in investigative studies or to the clinic requires its validation in terms of sensitivity, specificity, reproducibility, and correlation of the measurements with disease status. The validity of exhaled breath condensate as a tool for the assessment of airway oxidative stress is still questionable because of limitations in the reproducibility of analyzed oxidative biomarkers, with respect to both intra- and interindividual variability Quantification of chemical markers of protein oxidation in human urine, plasma, and other biological fluids also may identify pathways that promote oxidative stress in vivo and might indicate the extent of protein damage if these markers are liberated by proteolysis and excreted into such fluids Di-Tyr and NO 2 -Tyr are promising markers for these purposes because they are excreted in the urine in near quantitative yields and are not generated ex vivo during analysis by isotope-dilution GC-MS These observations raise the possibility that assaying oxidized amino acids in urine and plasma might be a useful approach to monitoring oxidative stress for human clinical and epidemiologic studies. Development of new biomarkers based on disease-induced protein modifications is limited by the lack of easy access to tissues from patient populations; therefore, the majority of discovery work will need to be carried out in animal models. The advantage provided by animal models is the ability to control and define disease stages; correlating these model diseases to actual patient populations has been difficult, however. As the technology develops to allow higher throughput screening, these candidate markers can be more easily tested against large groups. The successful development of effective antioxidant therapies remains a key goal, the attainment of which is required to elucidate the role played by accumulation of toxic oxidized molecules in the clinical picture of diseases associated with oxidative stress. The use of biomarkers provides a logical scientific basis for major intervention trials of antioxidants; such trials could, in turn, eventually validate or disprove the biomarker concept. Any intervention trial that does take place should be accompanied by measurements of one or more relevant biomarkers at intervals during the study. If the endpoint of the trial is disease incidence or mortality, such studies could help to validate or disprove the biomarker concept. However, with regard to this matter, we must highlight the disappointing clinical evidence showing the failure of antioxidant therapy for oxidative stress-associated pathologies such as cardiovascular disease, coronary artery disease, AD, and COPD At best, use of antioxidants is controversial and the ideal antioxidant strategy is still unknown. Human diseases that were found to be associated with increased oxidative stress on the basis of potential biomarkers of oxidative damage. Chemical structures of NO 2 -Tyr, halogenated tyrosines, and di-Tyr. Chemical structures of protein carbonyls arising from direct oxidation of amino acid side chains. Shown are 2-pyrrolidone resulting from direct oxidation of Pro residues , glutamic semialdehyde resulting from direct oxidation of Arg and Pro residues , α-aminoadipic semialdehyde resulting from direct oxidation of Lys residues , and 2-aminoketobutyric acid resulting from direct oxidation of Thr residues. For simplicity, stereochemistries are not indicated. A biomarker is defined as a characteristic that is objectively measured and evaluated as an indicator of normal biological processes, pathogenic processes, or pharmacologic responses to a therapeutic intervention. The most intuitive use for a biomarker is to help diagnose disease. Ideally, biomarkers of oxidative stress for human studies would be measurable in specimens that can be collected relatively easily, such as blood or urine. To serve these purposes, however, an ideal biomarker of oxidative damage should fulfill several conditions see text and Fig. We are profoundly grateful to the unknown reviewers whose insightful and constructive suggestions inspired us to produce a better effort. Preparation of this review was supported in part by FIRST Fondo Interno Ricerca Scientifica e Tecnologica , University of Milan, and by Fondazione Monte dei Paschi di Siena. Halliwell B, Gutteridge JMC. Free radicals in biology and medicine : pp Oxford University Press New York. Halliwell B. Role of free radicals in the neurodegenerative diseases: therapeutic implications for antioxidant treatment. Stocker R, Keaney JF, Jr. Role of oxidative modifications in atherosclerosis. Dalle-Donne I, Giustarini D, Colombo R, Rossi R, Milzani A. Protein carbonylation in human diseases. Dalle-Donne I, Scaloni A, Giustarini D, Cavarra E, Tell G, Lungarella G, et al. Proteins as biomarkers of oxidative stress in diseases: the contribution of redox proteomics. Klaunig JE, Kamendulis LM. The role of oxidative stress in carcinogenesis. Halliwell B, Whiteman M. Measuring reactive species and oxidative damage in vivo and in cell culture: how should you do it and what do the results mean?. Finkel T, Holbrook NJ. Oxidants, oxidative stress and the biology of ageing. Finkel T. Oxidant signals and oxidative stress. Dedon PC, Tannenbaum SR. Reactive nitrogen species in the chemical biology of inflammation. Stadtman ER, Berlett BS. Reactive oxygen—mediated protein oxidation in aging and disease. Linton S, Davies MJ, Dean RT. Protein oxidation and ageing. Schoneich C. Mass spectrometry in aging research. Davies MJ, Fu S, Wang H, Dean RT. Stable markers of oxidant damage to proteins and their application in study of human disease. Winterbourn CC, Buss H. Protein carbonyl measurement by enzyme-linked immunosorbent assay. Methods Enzymol ; : Levine RL, Wehr N, Williams JA, Stadtman ER, Shacter E. Determination of carbonyl groups in oxidized proteins. Methods Mol Biol ; 99 : 15 Pryor WA. Bio-assays for oxidative stress status BOSS : pp Elsevier Science BV Amsterdam. Griffiths HR, Moller L, Bartosz G, Bast A, Bertoni-Freddari C, Collins A, et al. Giustarini D, Dalle-Donne I, Colombo R, Milzani A, Rossi R. Adaptation of the Griess reaction for detection of nitrite in human plasma. Free Radic Res ; 38 : Tarpey MM, Wink DA, Grisham MB. Methods for detection of reactive metabolites of oxygen and nitrogen: in vitro and in vivo considerations. Uchida K. Carini M, Aldini G, Facino RM. Mass spectrometry for detection of 4-hydroxy- trans nonenal HNE adducts with peptides and proteins. Cracowski JL, Durand T, Bessard G. Isoprostanes as a biomarker of lipid peroxidation in humans: physiology, pharmacology and clinical implications. Montuschi P, Barnes PJ, Roberts LJ. Isoprostanes: markers and mediators of oxidative stress. Histidine and lysine as targets of oxidative modification. Draper HH, Csallany AS, Hadley M. Urinary aldehydes as indicators of lipid peroxidation in vivo. Free Radic Biol Med ; 29 : Wilson R, Lyall K, Smyth L, Fernie CE, Riemersma RA. Free Radic Biol Med ; 32 : Dean RT, Fu S, Stocker R, Davies MJ. Biochemistry and pathology of radical-mediated protein oxidation. Davies MJ. The oxidative environment and protein damage. Grune T, Merker K, Sandig G, Davies KJA. Selective degradation of oxidatively modified protein substrates by the proteasome. Biochem Biophys Res Commun ; : Ischiropoulos H. Biological selectivity and functional aspects of protein tyrosine nitration. Requena JR, Levine RL, Stadtman ER. Recent advances in the analysis of oxidized proteins. Stadtman ER, Levine RL. Free radical-mediated oxidation of free amino acids and amino acid residues in proteins. Stadtman ER, Moskovitz J, Levine RL. Oxidation of methionine residues of proteins: biological consequences. Keck S, Nitsch R, Grune T, Ullrich O. J Neurochem ; 85 : Sitte N, Huber M, Grune T, Ladhoff A, Doecke WD, Zglinicki T, et al. FASEB J ; 14 : Grune T, Jung T, Merker K, Davies KJA. Berliner J, Heinecke JW. The role of oxidized lipoproteins in atherogenesis. Slatter DA, Bolton CH, Bailey AJ. The importance of lipid-derived malondialdehyde in diabetes mellitus. Heinecke JW. Oxidized amino acids: culprits in human atherosclerosis and indicators of oxidative stress. Yoneyama Y, Sawa R, Suzuki S, Doi D, Yoneyama K, Otsubo Y, et al. Relationship between plasma malondialdehyde levels and adenosine deaminase activities in preeclampsia. Clin Chim Acta ; : Wood LG, Gibson PG, Garg ML. Biomarkers of lipid peroxidation, airway inflammation and asthma. Barnham KJ, Masters CL, Bush AI. Neurodegenerative diseases and oxidative stress. Esterbauer H, Schaur RJ, Zollner H. Chemistry and biochemistry of 4-hydroxynonenal, malonaldehyde and related aldehydes. Free Radic Biol Med ; 11 : 81 Eckl PM, Ortner A, Esterbauer H. Genotoxic properties of 4-hydroxyalkenals and analogous aldehydes. Mutat Res ; : Parola M, Bellomo G, Robino G, Barrera G, Dianzani MU. Feng Z, Hu W, Tang MS. transHydroxynonenal inhibits nucleotide excision repair in human cells: a possible mechanism for lipid peroxidation-induced carcinogenesis. Proc Natl Acad Sci U S A ; : Siems W, Grune T. Intracellular metabolism of 4-hydroxynonenal. Uchida K, Stadtman ER. Modification of histidine residues in proteins by reaction with 4-hydroxynonenal. Proc Natl Acad Sci U S A ; 89 : Butterfield DA. Butterfield DA, Castegna A, Lauderback CM, Drake J. Neurobiol Aging ; 23 : Montine TJ, Neely MD, Quinn JF, Beal MF, Markesbery WR, Roberts LJ, et al. Williams TI, Lynn BC, Markesbery WR, Lovell MA. Neurobiol Aging June 30, ; [Epub ahead of print]. Wataya T, Nunomura A, Smith MA, Siedlak SL, Harris PL, Shimohama S, et al. High molecular weight neurofilament proteins are physiological substrates of adduction by the lipid peroxidation product hydroxynonenal. J Biol Chem ; : Liu Q, Smith MA, Avila J, DeBernardis J, Kansal M, Takeda A, et al. Alzheimer-specific epitopes of τ represent lipid peroxidation-induced conformations. Free Radic Biol Med ; 38 : Jenner P. Role of reactive aldehyde in cardiovascular diseases. Acrolein modifies apolipoprotein α—I in the human artery wall. Ann N Y Acad Sci ; : Rahman I, van Schadewijk AA, Crowther AJ, Hiemstra PS, Stolk J, MacNee W, et al. Am J Respir Crit Care Med ; : Barreiro E, de la Puente B, Minguella J, Corominas JM, Serrano S, Hussain SN, et al. Oxidative stress and respiratory muscle dysfunction in severe chronic obstructive pulmonary disease. Klein T, Neuhaus K, Reutter F, Nusing RM. Generation of 8-epi-prostaglandin F 2α in isolated rat kidney glomeruli by a radical-independent mechanism. Br J Pharmacol ; : Tsikas D, Schwedhelm E, Suchy MT, Niemann J, Gutzki FM, Erpenbeck VJ, et al. Divergence in urinary 8-iso-PGF 2α iPF 2α -III, F 2t -IsoP levels from gas chromatography-tandem mass spectrometry quantification after thin-layer chromatography and immunoaffinity column chromatography reveals heterogeneity of 8-iso-PGF 2α. Possible methodological, mechanistic and clinical implications. Free radicals are oxygen-containing molecules with an uneven number of electrons. This uneven number of electrons allows free radicals to react easily with other molecules. Free radicals can cause large chain chemical reactions in your body because they react so easily with other molecules. These reactions are called oxidation. They can be beneficial or harmful. Antioxidants are molecules that can donate an electron to a free radical without making themselves unstable. This causes the free radical to stabilize and become less reactive. Read on to learn how oxidative stress affects the body and how to manage and prevent this imbalance. Oxidation is a normal and necessary process that takes place in your body. When functioning properly, free radicals can help fight off pathogens. Pathogens lead to infections. When there are more free radicals present than can be kept in balance by antioxidants, the free radicals can start doing damage to fatty tissue, DNA, and proteins in your body. Proteins, lipids, and DNA make up a large part of your body, so that damage can lead to a vast number of diseases over time. These include:. Everyone produces some free radicals naturally in their body through processes like exercise or inflammation. However, there are things you can do to minimize the effects of oxidative stress on your body. The main thing you can do is to increase your levels of antioxidants and decrease your formation of free radicals. Eating five servings per day of a variety of fruits and vegetables is the best way to provide your body what it needs to produce antioxidants. Examples of fruits and vegetables include:. Other healthy lifestyle choices can also prevent or reduce oxidative stress. Here are some lifestyle choices that will help:. |
Latest news | Health Conditions Health Products Discover Tools Connect. Larger randomized trials are needed to obtain clear scientific evidence on the benefits or risks of antioxidant supplementation during cancer treatment. Villamena, FA. Perera, N. In a study , scientists found that oxidative stress limited the spread of melanoma cancer cells in mice. Determination of carbonyl groups in oxidized proteins. |
Oxidative Stress and Disease | LC-MS—based stresa without derivatization may offer a oxiadtive to oxidative stress and disease problems. Fang, Y. Aust N Oxidative stress and disease J Ophthalmol disezse. Ballatori, N, Krance, SM, Notenboom, S, Shi, S, Tieu, K, Hammond, CL. In the blood vessel wall, all layers can produce ROS under pathological conditions, and most of them are primarily derived from NOX Reid, |
MDA, HNE, and Acrolein | Oxidative stress occurs naturally and plays a role in the aging process. A large body of scientific evidence suggests that long-term oxidative stress contributes to the development in a range of chronic conditions. Such conditions include cancer , diabetes , and heart disease. In this article, we explore what oxidative stress is, how it affects the body, and how to reduce it. Oxidative stress can occur when there is an imbalance of free radicals and antioxidants in the body. However, cells also produce antioxidants that neutralize these free radicals. In general, the body is able to maintain a balance between antioxidants and free radicals. Several factors contribute to oxidative stress and excess free radical production. These factors can include:. This type of oxidative stress causes mild inflammation that goes away after the immune system fights off an infection or repairs an injury. Uncontrolled oxidative stress can accelerate the aging process and may contribute to the development of a number of conditions. To discover more evidence-based information and resources for healthy aging, visit our dedicated hub. Free radicals, including reactive oxygen species, are molecules with one or more unpaired electron. Examples of free radicals include:. Cells contain small structures called mitochondria, which work to generate energy in the form of adenosine triphosphate ATP. Mitochondria combine oxygen and glucose to produce carbon dioxide, water, and ATP. Free radicals arise as byproducts of this metabolic process. External substances, such as cigarette smoke, pesticides, and ozone, can also cause the formation of free radicals in the body. Antioxidants are substances that neutralize or remove free radicals by donating an electron. The neutralizing effect of antioxidants helps protect the body from oxidative stress. Examples of antioxidants include vitamins A, C, and E. Like free radicals, antioxidants come from several different sources. Cells naturally produce antioxidants such as glutathione. Foods such as fruits and vegetables provide many essential antioxidants in the form of vitamins and minerals that the body cannot create on its own. The effects of oxidative stress vary and are not always harmful. For example, oxidative stress that results from physical activity may have beneficial, regulatory effects on the body. Exercise increases free radical formation, which can cause temporary oxidative stress in the muscles. However, the free radicals formed during physical activity regulate tissue growth and stimulate the production of antioxidants. Mild oxidative stress may also protect the body from infection and diseases. In a study , scientists found that oxidative stress limited the spread of melanoma cancer cells in mice. This can contribute to aging and may play an important role in the development of a range of conditions. Immune cells called macrophages produce free radicals while fighting off invading germs. These free radicals can damage healthy cells, leading to inflammation. Under normal circumstances, inflammation goes away after the immune system eliminates the infection or repairs the damaged tissue. Oxidative stress has a dual character: it is both harmful and beneficial to the body, because some ROS are signaling molecules on cellular signaling pathways. Lowering the level of oxidative stress through antioxidant supplements is therefore not beneficial in such cases Ye et al. Antioxidants are also prone to oxidation since oxidation and reduction reactions do not happen in isolation. AA, a potent antioxidant, mediates several physiological responses. This reaction is responsible for oxidative stress-produced DNA damage. However, the role of AA as anti- or pro-oxidant depends on the dose used, as observed in the case of ischemia-induced oxidative stress Seo and Lee, With increased oxygen tension, carotenoids tend to lose their antioxidant potential. Otherwise, α-tocopherol, a powerful antioxidant, becomes pro-oxidant at high concentrations Cillard and Cillard, Interestingly, when it reacts with a free radical, it becomes a radical in itself. If there is not enough AA for its regeneration, it will remain in that highly reactive state Lü et al. Flavonoids can also act as pro-oxidants depending on the concentrations used Prochazkova et al. Nevertheless, the extent to which these phytochemicals are capable of acting as anti- or pro-oxidants in vivo is still poorly understood, and this topic undoubtedly requires further research. The hypothesis that antioxidants could protect against cancer because they can neutralize reactive oxygen species ROS that can damage DNA has long been issued. In laboratory and animal studies, the presence of elevated levels of exogenous antioxidants has been shown to prevent the types of free radicals that have been associated with the development of cancer. A few randomized studies evaluating the role of antioxidant supplements for cancer prevention were conducted in collaboration with the National Cancer Institute Goodman et al. No data were obtained to justify that they are effective in primary cancer prevention. An analysis in the United States concluded that there is no clear scientific evidence for the benefits of vitamin and mineral supplements in cancer prevention. It is important to point out that there have been cases where people who have resorted to these types of supplements have encountered an unfavorable evolution of the disease. Preclinical studies also report that antioxidants have contributed to the expansion of tumor processes in animal models. A well-known case is that of vitamin A, for which the administration of high doses in supplements has been associated with an increased risk of cancer. Vitamin A can be obtained preformed from animal sources or plant products, derived from β-carotene. β-Carotene is an orange pigment found in fruits and vegetables carrots, sweet potatoes, mangoes, apricots , and in the body it is converted to vitamin A. A normal intake has a beneficial effect against the risk of cancer. However, studies have shown a correlation between the administration of β-carotene supplements and the risk of bladder cancer, as well as the risk of lung cancer in smokers Lin et al. In another study, the administration of α-tocopherol and β-carotene for lung cancer did not change the incidence of lung cancer. However, α-tocopherol supplements have been shown to be effective in prostate cancer whose incidence is reduced Goodman et al. A trial evaluated the effectiveness of long-term supplementation with vitamin E and vitamin C in the risk of developing cancer. One of the findings of the study was that these types of supplements do not reduce the risk of prostate cancer or the overall risk of cancer in men of middle age or older. No significant results were obtained regarding the risk of colorectal or lung cancer Gaziano et al. Vitamin E and C supplements showed poor results in many studies. There was a reduction in cardiovascular mortality, but no significant effect was observed on overall mortality. The authors concluded that vitamin E supplementation for the prevention of cardiovascular disease among healthy women is not justified. Moreover, cancer mortality is not significantly influenced by vitamin E supplementation Lee et al. The Selenium and Vitamin E Cancer Prevention Trial SELECT which included over 35, men over the age of 50, showed that selenium and vitamin E supplements do not prevent prostate cancer. This article summarizes the evidence from a large number of meta-analyzes covering the pathophysiological impact of antioxidants on the most common chronic diseases. The main criticism of the review is that the data were extracted from meta-analyzes and not from individual studies, but this can be considered an advantage because meta-analyzes provide the highest degree of evidence. In the case of antioxidants, studies show that more does not necessarily mean better. Consuming superfoods does not compensate for other unhealthy eating habits or an unbalanced lifestyle. Free radicals, as well as antioxidants, can have beneficial effects on the body. Therefore, we are talking about a balance and not a negative role attributed to free radicals and a positive one to antioxidants. Degradation of nucleic acids, proteins, lipids or other cellular components are among the effects that an excessive concentration of free radicals can generate. Risk factors leading to free radicals include air pollution, ionizing radiation, prolonged exercise, infections, excessive consumption of polyunsaturated fatty acids Poprac et al. On the other hand, antioxidants are considered to be the solution to these problems — substances that neutralize free radicals. In some situations, some substances act as antioxidants, in other situations they become prooxidants, depending on the chemical composition of the environment in which they are. There are many types of antioxidants, and the role in the body and the mechanisms by which they act are different. One misconception is that one antioxidant can be replaced with another, having the same effect. In fact, each has its own unique biological properties Chen X. There is also a significant difference between taking antioxidants from food and administering an isolated substance as a supplement. Many substances that demonstrate beneficial effects in the laboratory do not work when introduced into the human body. Many antioxidants do not have good bioavailability. The concentration of antioxidants such as polyphenols is sometimes so low in the blood that no significant effect is observed Fernández-García et al. Fruits and vegetables contain bioactive substances that in many cases do not work as antioxidants if we consider them outside of the body. But they work as antioxidants when they are in the body, because they activate their own antioxidant mechanisms. These bioactive substances are the secret behind vegetable consumption Kurutas, Antioxidant supplements may have different health benefits. On the one hand, it is possible that other substances present in food are responsible for the positive effects on health, not necessarily a certain type of antioxidant, but the synergistic effect of several substances. On the other hand, the chemical structure of antioxidants in food is often different from that identified in supplements. An example is vitamin E. There are eight variants of vitamin E in the foods we eat, while the supplements used in most studies contain only one form Firuzi et al. Studies also frequently include healthy people, for whom oxidative stress on the body is not significant to determine a risk of disease. Antioxidants can benefit certain categories of patients in whom there is a real, documented imbalance, but it may not bring anything extra for a person who gets a sufficient amount of nutrients from their diet. Observational studies analyze the trends, or habits of certain large population groups. In many, all the risk factors that could influence the course of the study can be controlled, and demonstrating a cause-effect relationship is difficult. We also cannot rely on small studies, carried out over a short period of time and using very concentrated substances extracted from different plant or animal products, to say that we have a superfood. Nutrition is a complex science, and at the moment we can only rely on the evidence accumulated so far. A food rich in antioxidants will not compensate for an unhealthy lifestyle. Oxidative stress can be reduced by approaching a balanced lifestyle. Nutrition plays a critical role, and the best treatment against oxidative stress is antioxidants. Oxidative stress plays an important role in the pathogenesis of potentially severe conditions. In the long term, increasing the level of prooxidant factors can cause structural defects in mitochondrial DNA and alterations in enzymatic functionality or cellular structures, with the appearance of functional, structural abnormalities or aberrations in gene expression. It has also been shown that in addition to metabolic products, other external agents can have a prooxidant effect, which has led to the conclusion that lifestyle and diet can play an important role in controlling oxidative stress. Plant-derived bioactive molecules have gained pivotal attention in recent years, given their therapeutic relevance in both disease prevention and treatment, whether using the whole plants, plant extracts or even the isolated constituents with full phytochemical profiles. The daily intake of a wide variety of phytochemicals has shown to be chemopreventive. It might hold promise for add-on treatment for several diseases, including cancer, diabetes, cardiovascular disease and neurodegenerative disorders. Larger randomized trials are needed to obtain clear scientific evidence on the benefits or risks of antioxidant supplementation during cancer treatment. Antioxidants are also prone to oxidation, and therefore their use as foods or supplements should be carefully considered because oxidation and reduction reactions do not happen in isolation. The intake of high doses of antioxidants has been increasingly highlighted since there is increasing evidence of some detrimental effects. The study of their chemical components as future prophylactic and therapeutic agents would be of particular interest, as they are more effective and safer than those widely available. In conclusion, oxidative stress is an important pathogenetic link for humans and studies in this field may be important elements in the future, to better understand and manage various diseases. JS-R and MS-R contributed to the conceptualization. NA, PZ, EV, and LD contributed to the validation investigation. EP, JR, PT, EA, IP, YE, and MB contributed to the resources. AP, MN, and AD: data curation. MS-R, AD, LP, MI, NM, MM, WS, DC, WC, and JS-R contributed to the review and editing. All authors contributed to the writing of the manuscript. All authors read and approved the final manuscript and contributed equally to the manuscript. The authors declare that the research was conducted in the absence of any commercial or financial relationships that could be construed as a potential conflict of interest. NM would like to thank the Portuguese Foundation for Science and Technology FCT—Portugal for the Strategic project ref. Abramov, A. Expression and modulation of an NADPH oxidase in mammalian astrocytes. doi: PubMed Abstract CrossRef Full Text Google Scholar. Alfonso-Prieto, M. The molecular mechanism of the catalase reaction. Aminjan, H. Life Sci. Andreyev, A. Mitochondrial metabolism of reactive oxygen species. Biochemistry 70, — Google Scholar. Antonioni, A. Redox homeostasis in sport: do athletes really need antioxidant support? Sports Med. Antunes dos Santos, A. Oxidative stress in methylmercury-induced cell toxicity. Toxics Aroor, A. Mitochondria and oxidative stress in the cardiorenal metabolic syndrome. Cardiorenal Med. Ayala, A. Lipid peroxidation: production, metabolism, and signaling mechanisms of malondialdehyde and 4-hydroxynonenal. Banafsheh, A. Studies on oxidants and antioxidants with a brief glance at their relevance to the immune system. Barbieri, R. Phytochemicals for human disease: An update on plant-derived compounds antibacterial activity. Barrera, G. Oxidative stress and lipid peroxidation products in cancer progression and therapy. ISRN Oncol. Bartosz, G. Reactive oxygen species: destroyers or messengers? Battelli, M. Pathophysiology of circulating xanthine oxidoreductase: new emerging roles for a multi-tasking enzyme. Acta , — Xanthine oxidoreductase in atherosclerosis pathogenesis: not only oxidative stress. Atherosclerosis , — Battin, E. Antioxidant activity of sulfur and selenium: a review of reactive oxygen species scavenging, glutathione peroxidase, and metal-binding antioxidant mechanisms. Cell Biochem. Bedard, K. The NOX family of ROS-generating NADPH oxidases: physiology and pathophysiology. Rev 87, — Benfeitas, R. New challenges to study heterogeneity in cancer redox metabolism. Cell Dev. Beyer, C. Antioxidant properties of melatonin—an emerging mystery. CrossRef Full Text Google Scholar. Bhattacharyya, A. Oxidative stress: an essential factor in the pathogenesis of gastrointestinal mucosal diseases. Birben, E. Oxidative stress and antioxidant defense. World Allergy Organ. Blokhuis, A. Protein aggregation in amyotrophic lateral sclerosis. Acta Neuropathol. Borek, C. Antioxidant health effects of aged garlic extract. Buga, A. Molecular and cellular stratagem of brain metastases associated with melanoma. Buj, R. Deoxyribonucleotide triphosphate metabolism in cancer and metabolic disease. Cadet, J. Formation and repair of oxidatively generated damage in cellular DNA. Free Radic. Oxidatively generated complex DNA damage: tandem and clustered lesions. Cancer Lett. DNA base damage by reactive oxygen species, oxidizing agents, and UV radiation. Cold Spring Harb. Cardoso, B. Glutathione peroxidase 4: A new player in neurodegeneration? Psychiatry 22, — Chen, J. Nitric oxide bioavailability dysfunction involves in atherosclerosis. Chen, X. Effect of puerarin in promoting fatty acid oxidation by increasing mitochondrial oxidative capacity and biogenesis in skeletal muscle in diabetic rats. Diabetes 8, 1— Oxidative stress in neurodegenerative diseases. Chondrogianni, N. Proteasome activation delays aging in vitro and in vivo. Cillard, J. Clark, I. Free radical-induced pathology. Cobley, J. Redox Biol. Conti, V. Antioxidant supplementation in the treatment of aging-associated diseases. Cortat, B. The relative roles of DNA damage induced by UVA irradiation in human cells. Curi, R. Regulatory principles in metabolism-then and now. Da Pozzo, E. Antioxidant and antisenescence effects of bergamot juice. Danielson, S. Davalli, P. ROS, cell senescence, and novel molecular mechanisms in aging and age-related diseases. De Bont, R. Endogenous DNA damage in humans: a review of quantitative data. Mutagenesis 19, — Delcambre, S. Buhlman Cham: Springer. Di Meo, S. Role of ROS and RNS sources in physiological and pathological conditions. Docea, A. Food Chem. Immunohistochemical expression of TGF beta TGF-β , TGF beta receptor 1 TGFBR1 , and Ki67 in intestinal variant of gastric adenocarcinomas. Duarte, T. Review: when is an antioxidant not an antioxidant? A review of novel actions and reactions of vitamin C. Egea, J. European contribution to the study of ROS: a summary of the findings and prospects for the future from the COST action BM EU-ROS. Elahi, M. Oxidative stress as a mediator of cardiovascular disease. Ertani, A. Biological activity of vegetal extracts containing phenols on plant metabolism. Molecules Esper, R. Endothelial dysfunction: a comprehensive appraisal. Fan, J. Quantitative flux analysis reveals folate-dependent NADPH production. Nature , — Fenga, C. Fernández-García, E. Carotenoids bioavailability from foods: from plant pigments to efficient biological activities. Food Res. Finkel, T. Oxidant signals and oxidative stress. Cell Biol. Signal transduction by reactive oxygen species. Oxidants, oxidative stress and the biology of ageing. Firuzi, O. Antioxidant therapy: current status and future prospects. Forcados, G. Oxidative stress and carcinogenesis: potential of phytochemicals in breast cancer therapy. Cancer 69, — Forman, H. Reactive oxygen species and cell signaling: respiratory burst in macrophage signaling. Care Med. Forni, C. Beneficial role of phytochemicals on oxidative stress and age-related diseases. BioMed Res. Fountoucidou, P. A mixture of routinely encountered xenobiotics induces both redox adaptations and perturbations in blood and tissues of rats after a long-term low-dose exposure regimen: The time and dose issue. Galati, G. Potential toxicity of flavonoids and other dietary phenolics: significance for their chemopreventive and anticancer properties. Gandhi, S. Mechanism of oxidative stress in neurodegeneration. Gaziano, J. Jama , 52— Gebicka, L. Catalytic scavenging of peroxynitrite by catalase. Glasauer, A. Targeting antioxidants for cancer therapy. Goodman, M. Clinical trials of antioxidants as cancer prevention agents: past, present, and future. Grigoras, A. Catalase immobilization—A review. Gutteridge, J. Free radicals and antioxidants in the year A historical look to the future. Hamanaka, R. Mitochondrial reactive oxygen species promote epidermal differentiation and hair follicle development. Hare, J. Hasanuzzaman, M. Glutathione in plants: biosynthesis and physiological role in environmental stress tolerance. Plants 23, — He, F. Redox roles of reactive oxygen species in cardiovascular diseases. Hernández-Almanza, A. Lycopene: progress in microbial production. Trends Food Sci. Herrera, B. Source of early reactive oxygen species in the apoptosis induced by transforming growth factor-beta in fetal rat hepatocytes. Homem de Bittencourt, P. Hsu, T. Activator protein 1 AP-1 - and nuclear factor kappaB NF-kappaB -dependent transcriptional events in carcinogenesis. Hu, N. Reactive oxygen species regulate myocardial mitochondria through post-translational modification. Species 2, — Huai, J. Structural properties and interaction partners of familial ALS-associated SOD1 mutants. Hussain, T. Oxidative stress and inflammation: what polyphenols can do for us? Imam, M. Antioxidants mediate both iron homeostasis and oxidative stress. Nutrients Jan, A. Heavy metals and human health: mechanistic insight into toxicity and counter defense system of antioxidants. Jaramillo, M. The emerging role of the Nrf2-Keap1 signaling pathway in cancer. Genes Dev. Jerome-Morais, A. Dietary supplements and human health: for better or for worse? Jomova, K. Advances in metal-induced oxidative stress and human disease. Toxicology , 65— Kabe, Y. Redox regulation of NF-kappaB activation: distinct redox regulation between the cytoplasm and the nucleus. Kaminski, K. Kang, Y. Chen Y, and epstein PN. Suppression of doxorubicin cardiotoxicity by overexpression of catalase in the heart of transgenic mice. Karam, B. Oxidative stress and inflammation as central mediators of atrial fibrillation in obesity and diabetes. Kimura, S. Black garlic: a critical review of its production, bioactivity, and application. Food Drug Anal. Klein, E. Vitamin E and the risk of prostate cancer: the Selenium and Vitamin E Cancer Prevention Trial SELECT. Jama , — Kocot, J. Does vitamin C influence neurodegenerative diseases and psychiatric disorders? Kostoff, R. Adverse health effects of 5G mobile networking technology under real-life conditions. Kucukgoncu, S. Alpha-lipoic acid ALA as a supplementation for weight loss: results from a meta-analysis of randomized controlled trials. Kumar, S. Chemistry and biological activities of flavonoids: an overview. World J. Kurutas, E. Lamy, M. Vincent Berlin: Springer , 83— Lazzarino, G. Water- and fat-soluble antioxidants in human seminal plasma and serum of fertile males. Antioxidants Lee, I. Jama , 56— Lee, S. Jiang, W. Aberrant expression of 5-lipoxygenase-activating protein 5-LOXAP has prognostic and survival significance in patients with breast cancer. Prostaglandins Leukot. Acids 74 , — Chan, H. Elevated levels of oxidative stress markers in exhaled breath condensate. Matsui, A. Cancer Lett. Ohtake, S. Oxidative stress marker 8-hydroxyguanosine is more highly expressed in prostate cancer than in benign prostatic hyperplasia. An, A. Association between expression of 8-OHdG and cigarette smoking in non-small cell lung cancer. Chakraborty, R. Systemic Inflammatory Response Syndrome StatPearls Ware, L. Shock 36 , 12—17 Alonso de Vega, J. Oxidative stress in critically ill patients with systemic inflammatory response syndrome. Bahar, I. Increased DNA damage and increased apoptosis and necrosis in patients with severe sepsis and septic shock. Care 43 , — Carpenter, C. Exhaled breath condensate isoprostanes are elevated in patients with acute lung injury or ARDS. Chest , — Sittipunt, C. Nitric oxide and nitrotyrosine in the lungs of patients with acute respiratory distress syndrome. Webber, R. Inducible nitric oxide synthase in circulating microvesicles: discovery, evolution, and evidence as a novel biomarker and the probable causative agent for sepsis. Joseph, L. Inhibition of NADPH oxidase 2 NOX2 prevents sepsis-induced cardiomyopathy by improving calcium handling and mitochondrial function. JCI Insight 2 , e Galley, H. Xanthine oxidase activity and free radical generation in patients with sepsis syndrome. Crouser, E. Endotoxin-induced mitochondrial damage correlates with impaired respiratory activity. Schorah, C. Total vitamin C, ascorbic acid, and dehydroascorbic acid concentrations in plasma of critically ill patients. Takeda, K. Plasma lipid peroxides and alpha-tocopherol in critically ill patients. Lyons, J. Cysteine metabolism and whole blood glutathione synthesis in septic pediatric patients. Granger, D. Role of xanthine oxidase and granulocytes in ischemia-reperfusion injury. This article documents the sources of oxidative stress in IRI. CAS PubMed Google Scholar. Matsushima, S. Physiological and pathological functions of NADPH oxidases during myocardial ischemia-reperfusion. Trends Cardiovasc. Duilio, C. Neutrophils are primary source of O2 radicals during reperfusion after prolonged myocardial ischemia. Heart Circ. Delanty, N. A potential quantitative marker of oxidant stress in vivo. Circulation 95 , — Reilly, M. Increased formation of the isoprostanes IPF2alpha-I and 8-epi-prostaglandin F2alpha in acute coronary angioplasty: evidence for oxidant stress during coronary reperfusion in humans. Circulation 96 , — Seet, R. Oxidative damage in ischemic stroke revealed using multiple biomarkers. Stroke 42 , — Nagayoshi, Y. Gong, P. Multiple basic-leucine zipper proteins regulate induction of the mouse heme oxygenase-1 gene by arsenite. Kronke, G. Expression of heme oxygenase-1 in human vascular cells is regulated by peroxisome proliferator-activated receptors. Peng, Z. Inhibitor of kappaB kinase beta regulates redox homeostasis by controlling the constitutive levels of glutathione. Rojo, A. Mulcahy, R. This article describes an essential role for NRF2 in GSH biosynthesis. Cuadrado, A. Therapeutic targeting of the NRF2 and KEAP1 partnership in chronic diseases. Drug Discov. This article provides a comprehensive review of NRF2 as a target for therapy. Moncada, S. Nitric oxide: physiology, pathophysiology, and pharmacology. This article provides a review of the primary role of nitric oxide in physiology and disease. Ursini, F. Redox homeostasis: the Golden Mean of healthy living. This article examines the relationship of redox homeostasis to disease. Pickering, A. Nrf2-dependent induction of proteasome and Pa28alphabeta regulator are required for adaptation to oxidative stress. Chowdhury, I. Oxidant stress stimulates expression of the human peroxiredoxin 6 gene by a transcriptional mechanism involving an antioxidant response element. Rusyn, I. Expression of base excision DNA repair genes is a sensitive biomarker for in vivo detection of chemical-induced chronic oxidative stress: identification of the molecular source of radicals responsible for DNA damage by peroxisome proliferators. Cancer Res. McCord, J. Superoxide dismutase: an enzymic function for erythrocuprein hemocuprein. Batinic-Haberle, I. Superoxide dismutase mimics: chemistry, pharmacology, and therapeutic potential. Bonetta, R. Potential therapeutic applications of MnSODs and SOD-mimetics. Chemistry 24 , — Faraggi, M. Chemical properties of water-soluble porphyrins. Pasternack, R. Catalysis of the disproportionation of superoxide by metalloporphyrins. Peretz, P. Chemical properties of water-soluble porphyrins 3. The reaction of superoxide radicals with some metalloporphyrins. Batinić-Haberle, I. Relationship among redox potentials, proton dissociation constants of pyrrolic nitrogens, and in vivo and in vitro superoxide dismutating activities of manganese iii and iron iii water-soluble porphyrins. Article Google Scholar. Jaramillo, M. Manganese iii meso-tetrakis N-ethylpyridiniumyl porphyrin acts as a pro-oxidant to inhibit electron transport chain proteins, modulate bioenergetics, and enhance the response to chemotherapy in lymphoma cells. Ferrer-Sueta, G. Reactions of manganese porphyrins with peroxynitrite and carbonate radical anion. An educational overview of the chemistry, biochemistry and therapeutic aspects of Mn porphyrins—from superoxide dismutation to H 2 O 2 -driven pathways. This article examines the potential use of SOD mimics in therapy. Rawal, M. Manganoporphyrins increase ascorbate-induced cytotoxicity by enhancing H 2 O 2 generation. Tetrahydrobiopterin rapidly reduces the SOD mimic Mn iii ortho-tetrakis N-ethylpyridiniumyl porphyrin. Design of Mn porphyrins for treating oxidative stress injuries and their redox-based regulation of cellular transcriptional activities. Amino Acids 42 , 95— Dorai, T. Amelioration of renal ischemia-reperfusion injury with a novel protective cocktail. Huber, W. Orgotein— bovine Cu-Zn superoxide dismutase , an anti-inflammatory protein drug: discovery, toxicology and pharmacology. Menander-Huber, K. Orgotein superoxide dismutase : a drug for the amelioration of radiation-induced side effects. A double-blind, placebo-controlled study in patients with bladder tumours. Sanchiz, F. Prevention of radioinduced cystitis by orgotein: a randomized study. Nielsen, O. Orgotein in radiation treatment of bladder cancer. A report on allergic reactions and lack of radioprotective effect. Acta Oncol. Mackensen, G. Neuroprotection from delayed postischemic administration of a metalloporphyrin catalytic antioxidant. Gauter-Fleckenstein, B. Comparison of two Mn porphyrin-based mimics of superoxide dismutase in pulmonary radioprotection. Rabbani, Z. Moeller, B. Radiation activates HIF-1 to regulate vascular radiosensitivity in tumors: role of reoxygenation, free radicals, and stress granules. Cancer Cell 5 , — Piganelli, J. A metalloporphyrin-based superoxide dismutase mimic inhibits adoptive transfer of autoimmune diabetes by a diabetogenic T-cell clone. Diabetes 51 , — Ganesh, D. Impact of superoxide dismutase mimetic AEOL on the endothelin system of Fischer rats. PLoS ONE 11 , e Benatar, M. Lost in translation: treatment trials in the SOD1 mouse and in human ALS. Aston, K. Computer-aided design CAD of Mn ii complexes: superoxide dismutase mimetics with catalytic activity exceeding the native enzyme. Heer, C. Superoxide dismutase mimetic GC enhances the oxidation of pharmacological ascorbate and its anticancer effects in an H 2 O 2 -dependent manner. Antioxidants 7 , 18 Salvemini, D. Pharmacological manipulation of the inflammatory cascade by the superoxide dismutase mimetic, M Amelioration of joint disease in a rat model of collagen-induced arthritis by M, a superoxide dismutase mimetic. Arthritis Rheum. Masini, E. Protective effects of M, a selective superoxide dismutase mimetic, in myocardial ischaemia and reperfusion injury in vivo. Anderson, C. Doctrow, S. McDonald, M. A superoxide dismutase mimetic with catalase activity EUK-8 reduces the organ injury in endotoxic shock. This article examines the advantage of having catalase activity in SOD mimics. Xu, Y. Cardioprotection by chronic estrogen or superoxide dismutase mimetic treatment in the aged female rat. van Empel, V. EUK-8, a superoxide dismutase and catalase mimetic, reduces cardiac oxidative stress and ameliorates pressure overload-induced heart failure in the harlequin mouse mutant. Izumi, M. Superoxide dismutase mimetics with catalase activity reduce the organ injury in hemorrhagic shock. Shock 18 , — Jung, C. Chatterjee, P. EUK reduces renal dysfunction and injury caused by oxidative and nitrosative stress of the kidney. Baker, K. Langan, A. Liu, Z. Himori, K. PLoS ONE 12 , e Chronic antioxidant enzyme mimetic treatment differentially modulates hyperthermia-induced liver HSP70 expression with aging. Day, B. Catalase and glutathione peroxidase mimics. Ebselen, a selenoorganic compound as glutathione peroxidase mimic. Nakamura, Y. Ebselen, a glutathione peroxidase mimetic seleno-organic compound, as a multifunctional antioxidant. Implication for inflammation-associated carcinogenesis. Kil, J. Ebselen treatment reduces noise induced hearing loss via the mimicry and induction of glutathione peroxidase. Garland, M. The clinical drug ebselen attenuates inflammation and promotes microbiome recovery in mice after antibiotic treatment for CDI. Cell Rep. Singh, N. Effect of the putative lithium mimetic ebselen on brain myo-inositol, sleep, and emotional processing in humans. Neuropsychopharmacology 41 , — Ogawa, A. Ebselen in acute middle cerebral artery occlusion: a placebo-controlled, double-blind clinical trial. Saito, I. Neuroprotective effect of an antioxidant, ebselen, in patients with delayed neurological deficits after aneurysmal subarachnoid hemorrhage. Neurosurgery 42 , —; discussion — Yamaguchi, T. Ebselen in acute ischemic stroke: a placebo-controlled, double-blind clinical trial. Stroke 29 , 12—17 ICAM-1 and VCAM-1 expression induced by TNF-alpha are inhibited by a glutathione peroxidase mimic. Castagne, V. Blum, S. Haptoglobin genotype determines myocardial infarct size in diabetic mice. Puntarulo, S. Comparison of the ability of ferric complexes to catalyze microsomal chemiluminescence, lipid peroxidation, and hydroxyl radical generation. Brittenham, G. Efficacy of deferoxamine in preventing complications of iron overload in patients with thalassemia major. Raftos, J. Kinetics of uptake and deacetylation of N-acetylcysteine by human erythrocytes. Rushworth, G. Existing and potential therapeutic uses for N-acetylcysteine: the need for conversion to intracellular glutathione for antioxidant benefits. Smilkstein, M. Efficacy of oral N-acetylcysteine in the treatment of acetaminophen overdose. Analysis of the national multicenter study to Conrad, C. Long-term treatment with oral N-acetylcysteine: affects lung function but not sputum inflammation in cystic fibrosis subjects. A phase II randomized placebo-controlled trial. Xu, R. Effectiveness of N-acetylcysteine for the prevention of contrast-induced nephropathy: a systematic review and meta-analysis of randomized controlled trials. Heart Assoc. Wendel, A. The level and half-life of glutathione in human plasma. Anderson, M. Glutathione monoesters. Levy, E. Transport of glutathione diethyl ester into human cells. USA 90 , — This article demonstrates that glutathione diethyl ester is highly effective as a delivery agent for GSH in human cells to decrease oxidative stress. Puri, R. Transport of glutathione, as gamma-glutamylcysteinylglycyl ester, into liver and kidney. USA 80 , — Wellner, V. Radioprotection by glutathione ester: transport of glutathione ester into human lymphoid cells and fibroblasts. USA 81 , — Tsan, M. Modulation of endothelial GSH concentrations: effect of exogenous GSH and GSH monoethyl ester. CAS Google Scholar. Grattagliano, I. Reperfusion injury of the liver: role of mitochondria and protection by glutathione ester. Glutathione monoethyl ester provides neuroprotection in a rat model of stroke. Chen, T. Glutathione monoethyl ester protects against glutathione deficiencies due to aging and acetaminophen in mice. Ageing Dev. Glutathione monoethyl ester: preparation, uptake by tissues, and conversion to glutathione. Zeevalk, G. Howden, R. Nrf2 and cardiovascular defense. Cell Longev. Gan, L. Oxidative damage and the Nrf2-ARE pathway in neurodegenerative diseases. Acta , — Boutten, A. Protective role of Nrf2 in the lungs against oxidative airway diseases. Google Scholar. McMahon, M. Seo, H. PLoS ONE 15 , e Lee, D. The hypertension drug, verapamil, activates Nrf2 by promoting pdependent autophagic Keap1 degradation and prevents acetaminophen-induced cytotoxicity. BMB Rep. Li, J. Green tea extract provides extensive Nrf2-independent protection against lipid accumulation and NFkappaB pro-inflammatory responses during nonalcoholic steatohepatitis in mice fed a high-fat diet. Food Res. Pandurangan, A. Biofactors 41 , 1—14 Yagishita, Y. Broccoli or sulforaphane: is it the source or dose that matters? Molecules 24 , This article evaluates the current knowledge regarding bioavailability and efficacy of glucoraphanin and sulforaphane in terms of dose and route of administration. Robledinos-Anton, N. Activators and inhibitors of NRF2: a review of their potential for clinical development. This article reviewed electrophilic and non-electrophilic NRF2 activators in clinical trials for various chronic diseases including cancer. Kwon, J. Sulforaphane inhibits restenosis by suppressing inflammation and the proliferation of vascular smooth muscle cells. Atherosclerosis , 41—49 Shimizu, K. Anti-inflammatory action of curcumin and its use in the treatment of lifestyle-related diseases. de Sa Coutinho, D. Anti-inflammatory effects of resveratrol: mechanistic insights. Jiang, Z. Discovery and development of Kelch-like ECH-associated protein 1. Nuclear factor erythroid 2-related factor 2 KEAP1:NRF2 protein-protein interaction inhibitors: achievements, challenges, and future directions. Satoh, T. Recent advances in understanding NRF2 as a druggable target: development of pro-electrophilic and non-covalent NRF2 activators to overcome systemic side effects of electrophilic drugs like dimethyl fumarate. FRes 6 , This article evaluates NRF2 activators designed to avoid the systemic side effects caused by electrophilic activators. Couch, R. Studies on the reactivity of CDDO, a promising new chemopreventive and chemotherapeutic agent: implications for a molecular mechanism of action. This article demonstrates that conjugation of electrophilic cyanoenone compounds and KEAP1 is selective and reversable. Kostov, R. Pharmacokinetics and pharmacodynamics of orally administered acetylenic tricyclic bis cyanoenone , a highly potent Nrf2 activator with a reversible covalent mode of action. Dinkova-Kostova, A. An exceptionally potent inducer of cytoprotective enzymes: elucidation of the structural features that determine inducer potency and reactivity with Keap1. Sedlak, T. Sulforaphane augments glutathione and influences brain metabolites in human subjects: a clinical pilot study. Neuropsychiatry 3 , — PubMed PubMed Central Google Scholar. Wise, R. Lack of effect of oral sulforaphane administration on Nrf2 expression in COPD: a randomized, double-blind, placebo controlled trial. Greaney, A. Sulforaphane inhibits multiple inflammasomes through an Nrf2-independent mechanism. Roy, S. Satoh, H. Nrf2 prevents initiation but accelerates progression through the Kras signaling pathway during lung carcinogenesis. This article demonstrates that NRF2-deficient mice exhibited an increase in tumour foci after urethane induction but a reduction in tumours with more malignant characteristics. Wiel, C. BACH1 stabilization by antioxidants stimulates lung cancer metastasis. Cell , — e This article demonstrates that long-term supplementation with N -acetylcysteine and vitamin E promoted KRAS-driven lung cancer metastasis, and NRF2 inhibitor BACH1 stimulated glycolysis-dependent lung cancer metastasis in a mouse model. Tao, S. The effects of NRF2 modulation on the initiation and progression of chemically and genetically induced lung cancer. This article demonstrates that sulforaphane prevented the initiation of vinyl carbamate-induced lung cancer in mouse models but promoted the progression of pre-existing tumours. Shibata, T. Genetic alteration of Keap1 confers constitutive Nrf2 activation and resistance to chemotherapy in gallbladder cancer. Gastroenterology , — Homma, S. Nrf2 enhances cell proliferation and resistance to anticancer drugs in human lung cancer. Jiang, T. High levels of Nrf2 determine chemoresistance in type II endometrial cancer. Roh, J. Nrf2 inhibition reverses the resistance of cisplatin-resistant head and neck cancer cells to artesunate-induced ferroptosis. Sporn, M. NRF2 and cancer: the good, the bad and the importance of context. Cancer 12 , — Milkovic, L. Controversy about pharmacological modulation of Nrf2 for cancer therapy. Wu, S. Nrf2 in cancers: a double-edged sword. Cancer Med. Massart, C. Diphenyleneiodonium, an inhibitor of NOXes and DUOXes, is also an iodide-specific transporter. FEBS Open Bio. Augsburger, F. Pharmacological characterization of the seven human NOX isoforms and their inhibitors. |
0 thoughts on “Oxidative stress and disease”